How Technology is Changing Our Understanding of Physics
In an age where technology evolves at a breakneck pace, one field that has seen profound transformations is physics. Imagine stepping into a world where the mysteries of the universe are not just theoretical but tangible, thanks to cutting-edge technology. This article explores the transformative impact of technology on the field of physics, highlighting advancements that enhance research, experimentation, and our grasp of fundamental concepts. From the tiniest particles to the vast expanses of the cosmos, technology is reshaping our understanding and pushing the boundaries of what we thought was possible.
New technologies have revolutionized experimental physics, enabling more precise measurements and innovative methods that allow scientists to explore phenomena previously thought unreachable. For instance, the development of high-energy particle accelerators has opened doors to studying fundamental particles and forces that govern the universe. With these advancements, physicists can now conduct experiments that yield data with remarkable accuracy, leading to groundbreaking discoveries.
The rise of powerful computing has transformed theoretical physics, allowing for complex simulations and calculations that deepen our understanding of the universe's laws. Imagine being able to run thousands of simulations in a matter of hours, exploring scenarios that would take years to test physically. This capability is revolutionizing how physicists approach problems, enabling them to visualize complex interactions and phenomena that were once beyond reach.
Quantum computing offers unprecedented capabilities for simulating quantum systems, providing insights into particle behavior and interactions that classical computers cannot achieve. With quantum simulators, researchers can explore the intricacies of quantum mechanics, leading to potential breakthroughs in materials science, cryptography, and even medicine. The implications of this technology are vast, opening new avenues for understanding the fundamental building blocks of our universe.
Machine learning techniques are increasingly used to analyze vast datasets in physics, helping to uncover patterns and make predictions in complex systems. By leveraging algorithms that can learn from data, physicists can identify correlations and trends that would be impossible to discern manually. This approach not only accelerates research but also enhances the accuracy of predictions, paving the way for new discoveries.
The integration of big data analytics in experimental physics is leading to new discoveries, as researchers can now process and interpret data at unprecedented speeds. Imagine a physicist sifting through terabytes of data from experiments and simulations—what once took months can now be done in days. This capability allows scientists to focus on interpretation and innovation, rather than getting bogged down in data management.
Advanced modeling tools allow physicists to simulate astrophysical events, enhancing our understanding of cosmic phenomena such as black holes and supernovae. These simulations provide a virtual playground for researchers, enabling them to test theories and observe outcomes without the constraints of reality. The insights gained from these models can lead to significant advancements in our understanding of the universe.
Technology is reshaping physics education through interactive simulations and virtual labs, making complex concepts more accessible and engaging for students. Gone are the days when students had to rely solely on textbooks; now, they can visualize concepts in real-time, experiment virtually, and collaborate with peers worldwide. This shift not only enhances learning but also fosters a deeper appreciation for the beauty of physics.
The proliferation of online resources and courses has democratized access to physics education, enabling learners worldwide to study at their own pace. Whether you're a high school student curious about the laws of motion or a seasoned professional looking to brush up on quantum mechanics, the internet offers a plethora of resources. This accessibility is breaking down barriers and inspiring a new generation of physicists.
Virtual reality technology provides immersive learning experiences, allowing students to visualize and interact with physical concepts in a three-dimensional space. Imagine walking through a model of an atom or observing the effects of gravity in a simulated environment. These engaging experiences not only make learning fun but also help solidify complex concepts in students' minds.
Technological advancements foster collaborations across disciplines, leading to innovative approaches in physics research and applications in fields like engineering and medicine. By breaking down silos, physicists can work with biologists, chemists, and engineers to tackle complex problems that require a multifaceted approach. This interdisciplinary collaboration is where some of the most exciting advancements are occurring.
The intersection of physics and biology is yielding breakthroughs in understanding complex biological systems, driven by technological advancements in imaging and analysis. Techniques such as CRISPR gene editing and advanced imaging technologies are allowing researchers to explore the mechanics of life at an unprecedented level. This fusion of disciplines is not just expanding our knowledge; it's also leading to practical applications in medicine and biotechnology.
Technology is pivotal in addressing environmental challenges, enabling physicists to develop sustainable solutions through advanced modeling and data analysis techniques. With the help of sophisticated simulations and data analytics, researchers can predict climate patterns, assess the impact of human activities, and devise strategies for mitigating environmental damage. This proactive approach is essential for ensuring a sustainable future.
- How is technology impacting physics research?
Technology enhances experimental techniques, computational capabilities, and data analysis, leading to new discoveries and deeper understanding. - What role does machine learning play in physics?
Machine learning helps analyze large datasets, uncover patterns, and make predictions, accelerating research and improving accuracy. - How are online platforms transforming physics education?
They provide accessible resources, allowing learners to study at their own pace and engage with interactive simulations.
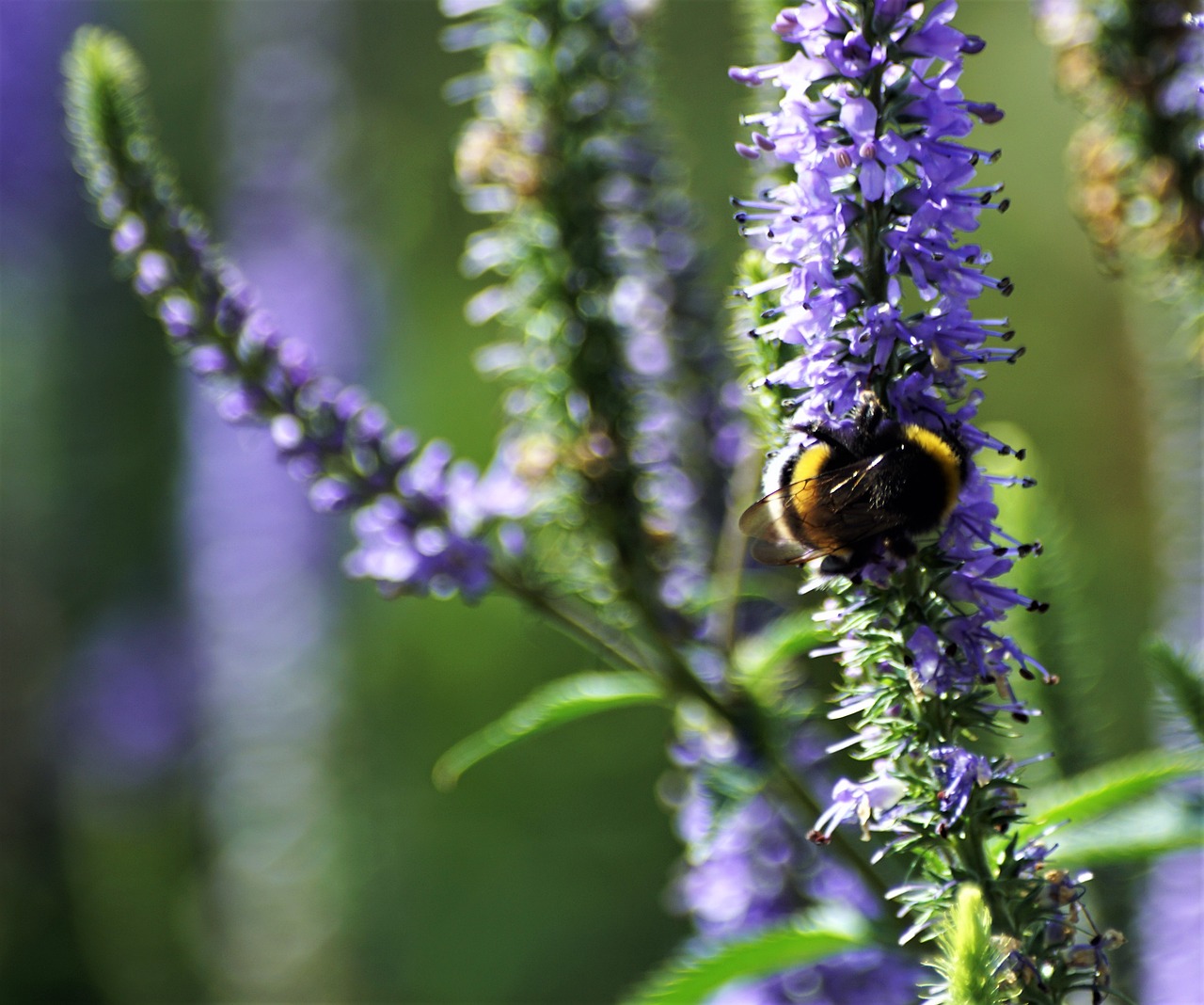
Advancements in Experimental Techniques
The realm of experimental physics has undergone a remarkable transformation, driven largely by technological advancements. Imagine a world where scientists can measure the tiniest particles with unprecedented accuracy—this is no longer a distant dream but a reality thanks to cutting-edge tools and techniques. In the past, experiments were often limited by the precision of the instruments available, but today, we stand on the shoulders of giants, utilizing technology that enhances our ability to explore the very fabric of the universe.
One of the most significant advancements is the development of high-precision measurement devices. These instruments, which include laser interferometers and atomic clocks, allow physicists to observe phenomena that were previously thought to be beyond our reach. For instance, gravitational waves, ripples in spacetime caused by massive cosmic events, were only detected after the installation of advanced laser interferometry technology. This breakthrough not only confirmed a century-old prediction by Einstein but also opened a new window into the universe, allowing us to 'hear' the cosmos in ways we never thought possible.
Furthermore, innovative experimental techniques such as particle accelerators have revolutionized our understanding of fundamental particles. These colossal machines propel particles to near-light speeds, enabling researchers to collide them and study the resulting interactions. The discoveries made at facilities like CERN have not only confirmed existing theories but also led to the identification of new particles, expanding our understanding of the universe's building blocks.
Another fascinating advancement is the use of quantum sensors. These devices exploit the principles of quantum mechanics to achieve sensitivity levels that classical sensors cannot match. For example, quantum sensors can detect minute changes in gravitational fields, which has implications for everything from geophysics to medical imaging. By harnessing the quirks of quantum mechanics, physicists are pushing the boundaries of what we can measure and observe.
Moreover, the integration of robotics and automation in experimental setups has streamlined the process of data collection and analysis. Automated systems can conduct experiments with minimal human intervention, increasing efficiency and reducing the likelihood of human error. This technological leap not only accelerates the pace of research but also allows scientists to focus on interpreting results rather than managing tedious manual tasks.
In addition to these advancements, the collaboration between physicists and engineers has led to the development of sophisticated simulation software that models complex physical systems. This software enables researchers to predict outcomes before conducting actual experiments, saving time and resources. As a result, scientists can design experiments more effectively, honing in on the most promising avenues of research.
In summary, the advancements in experimental techniques are nothing short of revolutionary. They have transformed the landscape of physics, allowing us to probe deeper into the mysteries of the universe. As technology continues to evolve, we can only imagine the discoveries that await us, pushing the boundaries of our understanding and reshaping our view of reality.
- What are some examples of advanced experimental techniques in physics?
Examples include high-precision measurement devices, particle accelerators, quantum sensors, and automated robotic systems. - How have particle accelerators changed our understanding of physics?
Particle accelerators allow scientists to collide particles at high speeds, leading to the discovery of new particles and confirming existing theories. - What role does technology play in modern physics experiments?
Technology enhances measurement precision, streamlines data collection, and enables complex simulations, significantly advancing our research capabilities.
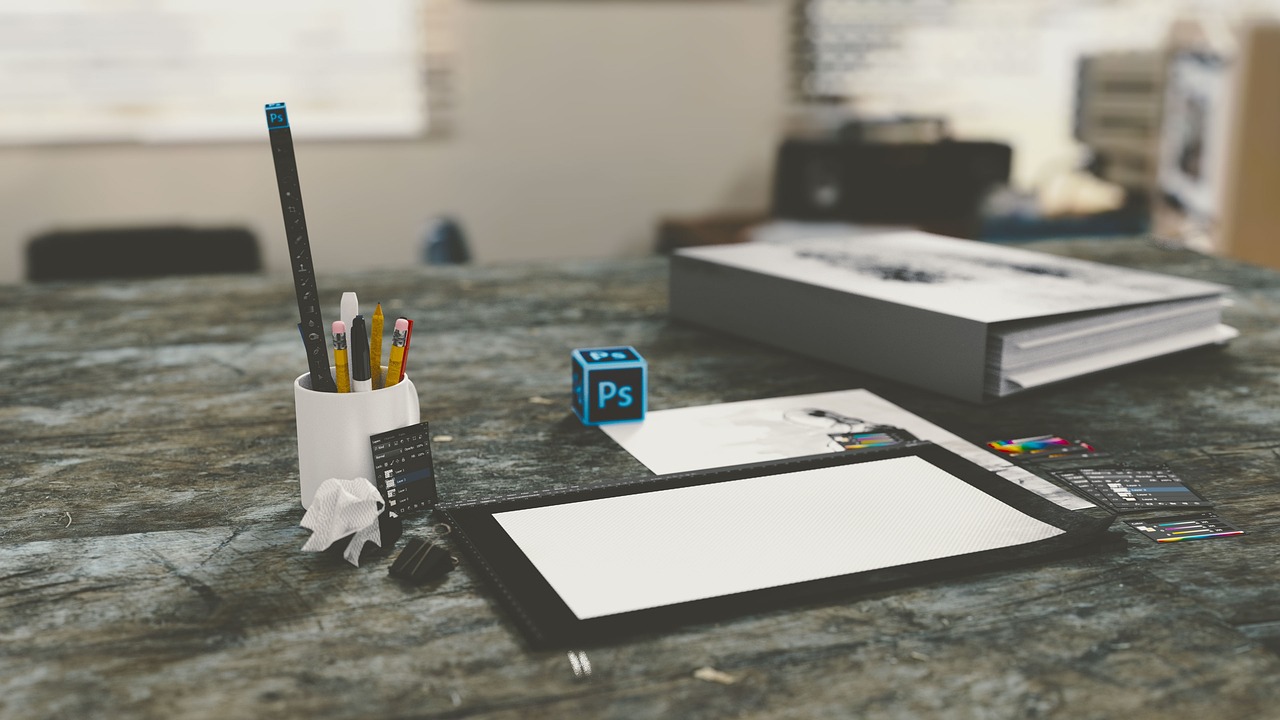
Computational Physics
In the realm of physics, the advent of powerful computing has been nothing short of a revolution. Imagine trying to understand the intricate dance of particles in the universe without the aid of advanced computational tools—it's like trying to navigate a maze blindfolded! With the rise of computational physics, scientists are now able to perform complex simulations and calculations that were once deemed impossible. This field not only deepens our understanding of the fundamental laws of the universe but also opens up new avenues for exploration.
One of the most exciting aspects of computational physics is its ability to simulate scenarios that are either too dangerous or impractical to recreate in a laboratory setting. For instance, consider the chaotic behavior of turbulent fluids or the mysterious interactions of subatomic particles. By utilizing sophisticated algorithms and high-performance computing, researchers can model these phenomena with remarkable accuracy, leading to insights that were previously out of reach.
Among the many breakthroughs in computational physics, quantum simulations stand out as a game-changer. Quantum computing harnesses the peculiar properties of quantum mechanics to perform calculations at speeds that classical computers can only dream of. This capability allows physicists to simulate quantum systems, providing unprecedented insights into particle behavior and interactions. For example, researchers can explore how particles entangle and influence one another, revealing the underlying principles that govern the quantum world.
As if that weren't enough, the integration of machine learning techniques into computational physics is transforming the way we analyze data. By employing algorithms that can learn from vast datasets, physicists are uncovering patterns and making predictions in complex systems. This marriage of physics and artificial intelligence not only accelerates research but also enhances our ability to make sense of intricate data landscapes. Imagine having a super-intelligent assistant that can sift through mountains of data to highlight the most critical findings—this is the power of machine learning in action!
The fusion of big data analytics with experimental physics is leading to groundbreaking discoveries. Researchers can now process and interpret data at unprecedented speeds, allowing them to identify trends and anomalies that would have been impossible to detect manually. For instance, in particle physics, the analysis of data from colliders like the Large Hadron Collider (LHC) has been greatly enhanced through computational techniques, enabling scientists to confirm the existence of new particles and phenomena. This data-driven approach is reshaping our understanding of the universe, one discovery at a time.
Advanced modeling tools are also making waves in the field of astrophysics. Physicists can simulate cosmic events such as black holes, supernovae, and even the formation of galaxies. These simulations not only help us visualize the vastness of the universe but also provide crucial insights into the mechanics of celestial phenomena. By comparing simulated results with observational data, scientists can refine their theories and deepen their understanding of the cosmos.
In summary, computational physics is a dynamic and rapidly evolving field that is reshaping our understanding of the universe. Through quantum simulations, machine learning applications, and advanced modeling techniques, physicists are uncovering the mysteries of nature at an unprecedented pace. As we continue to harness the power of technology, the possibilities for discovery are limitless.
- What is computational physics? - Computational physics is a branch of physics that uses computational methods and algorithms to solve complex physical problems and simulate physical systems.
- How does quantum computing impact physics? - Quantum computing allows physicists to perform simulations and calculations that are infeasible for classical computers, leading to deeper insights into quantum systems.
- What role does machine learning play in physics? - Machine learning helps physicists analyze large datasets, uncover patterns, and make predictions, enhancing the research process significantly.
- Can computational physics help with environmental issues? - Yes, computational physics can model environmental phenomena and help develop sustainable solutions by analyzing complex data related to climate and ecosystems.
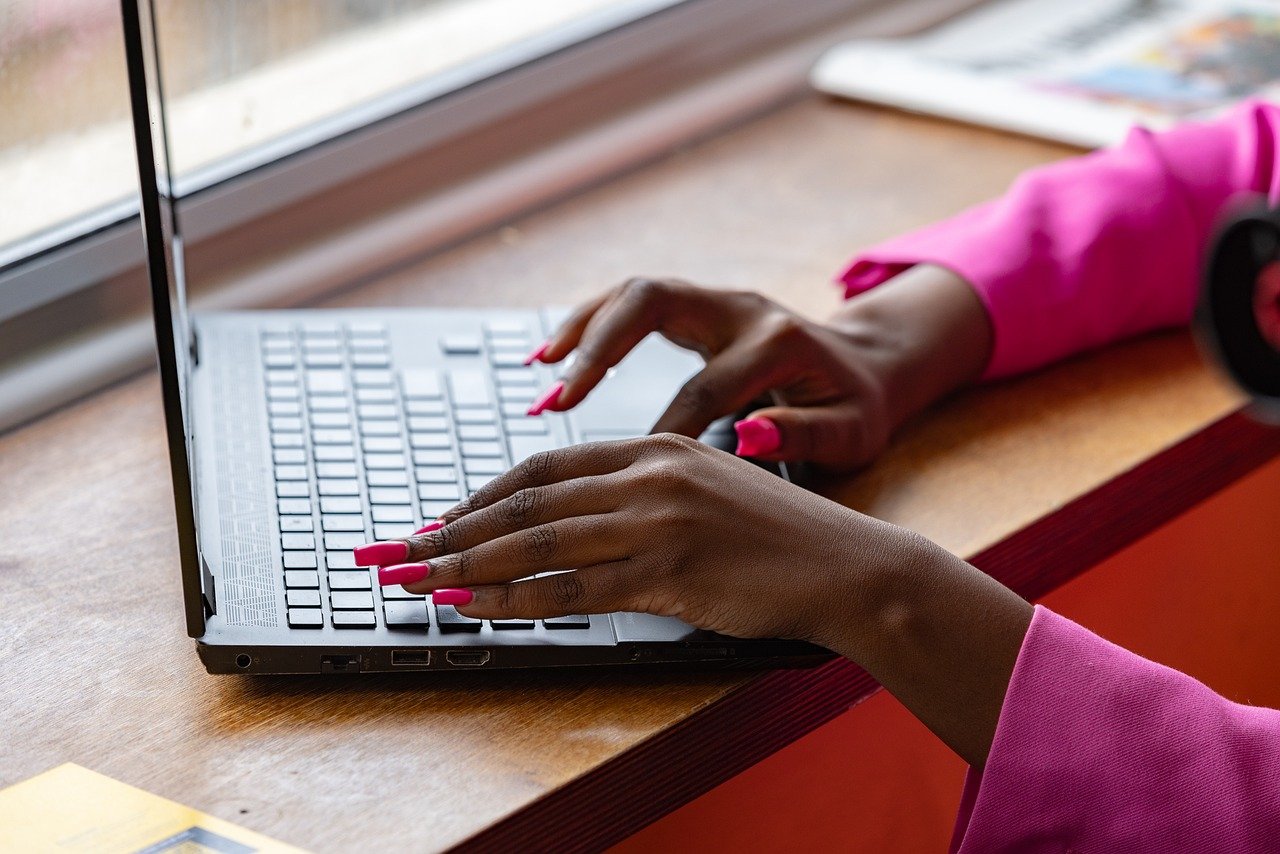
Quantum Simulations
Quantum simulations represent a groundbreaking frontier in the realm of physics, where traditional computing methods fall short in unraveling the complexities of quantum systems. Imagine trying to solve a puzzle with pieces that change shape every time you touch them; this is akin to the challenge faced by physicists when studying quantum particles. With the advent of quantum computing, researchers can now simulate these elusive systems with a level of precision that was once thought impossible.
At the heart of quantum simulations lies the ability to model interactions between particles in ways that classical computers simply cannot replicate. Classical computers operate on bits, the smallest unit of data, which can either be a 0 or a 1. In contrast, quantum computers utilize qubits, which can exist in multiple states simultaneously thanks to the principle of superposition. This property allows quantum computers to process vast amounts of information at unprecedented speeds, making them ideal for simulating complex quantum phenomena.
For instance, consider the simulation of quantum entanglement, a phenomenon where particles become intertwined and the state of one instantly influences the state of another, regardless of the distance separating them. This has profound implications for fields such as quantum cryptography and teleportation. By leveraging quantum simulations, scientists can explore the nuances of entangled states, paving the way for advancements in secure communication technologies.
Moreover, quantum simulations are not just theoretical; they have practical applications that could revolutionize various industries. Here are a few areas where quantum simulations are making a significant impact:
- Material Science: Simulating the properties of new materials at the atomic level can lead to the discovery of superconductors or more efficient batteries.
- Chemistry: Understanding chemical reactions and molecular interactions can accelerate drug discovery and development.
- Optimization Problems: Quantum simulations can help solve complex optimization problems in logistics, finance, and artificial intelligence.
As we stand on the brink of a quantum revolution, the implications of these simulations extend far beyond physics. They challenge our understanding of the universe and open doors to technologies that could redefine our daily lives. The potential is immense, and as researchers continue to harness the power of quantum computing, we can expect to witness a surge in discoveries that will reshape our scientific landscape.
In conclusion, quantum simulations are not just a niche area of research; they represent a paradigm shift in how we approach the mysteries of the quantum world. As technology continues to evolve, the synergy between quantum theory and computational power will undoubtedly lead to breakthroughs that we can only begin to imagine today.
- What are quantum simulations? Quantum simulations are computational methods that use quantum computing to model and analyze quantum systems, providing insights into their behavior and interactions.
- How do quantum simulations differ from classical simulations? Unlike classical simulations that rely on binary bits, quantum simulations utilize qubits, allowing them to perform calculations in parallel and handle complex quantum phenomena more effectively.
- What are the applications of quantum simulations? Quantum simulations have applications in material science, chemistry, optimization problems, and quantum cryptography, among others.
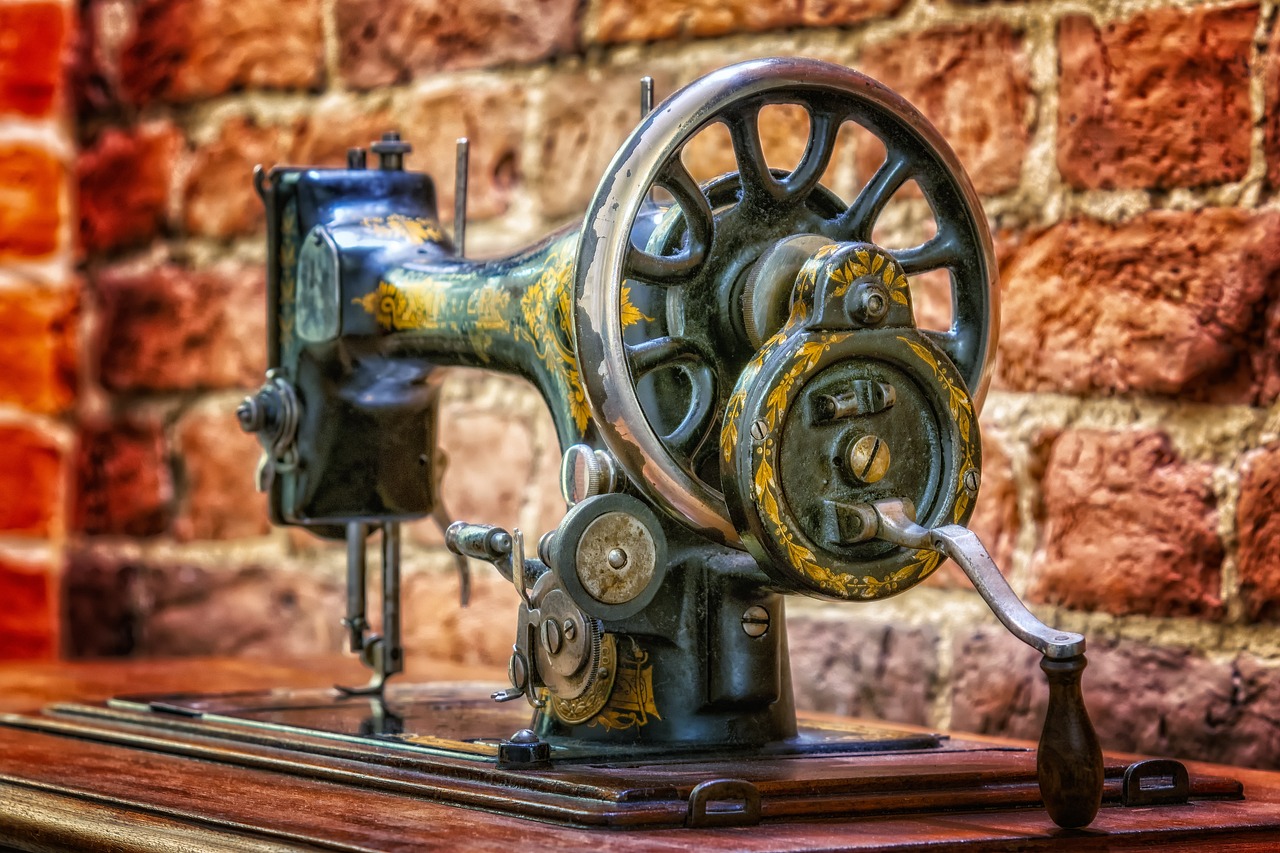
Machine Learning Applications
In the vast expanse of modern physics, machine learning is emerging as a game-changer, akin to how the telescope transformed our view of the cosmos. By employing sophisticated algorithms, physicists can sift through mountains of data, uncovering patterns that the human eye might miss. Imagine trying to find a needle in a haystack; machine learning acts like a magnet, attracting those elusive insights and making sense of complex systems.
One of the most exciting applications of machine learning in physics is its ability to enhance data analysis. With experiments generating more data than ever before, traditional methods of analysis are often inadequate. Machine learning algorithms can process these vast datasets at lightning speed, identifying correlations and anomalies that lead to new discoveries. For instance, in particle physics, machine learning techniques are deployed to analyze collision data from particle accelerators, helping scientists determine the existence of new particles and phenomena.
Moreover, machine learning isn't just about crunching numbers; it's about predictive modeling. These algorithms can learn from historical data to predict future outcomes, which is invaluable in fields like astrophysics. For example, researchers are using machine learning to predict the behavior of celestial bodies, such as the trajectory of asteroids or the dynamics of galaxy formation. This predictive capability allows scientists to simulate scenarios that would be impossible to test through direct observation.
To illustrate the impact of machine learning in physics, consider the following table that summarizes its key applications:
Application | Description |
---|---|
Data Classification | Sorting and categorizing data from experiments to identify significant patterns. |
Predictive Analytics | Using historical data to forecast future events in physical systems. |
Anomaly Detection | Identifying outliers in data that may indicate new phenomena or errors. |
Image Recognition | Analyzing images from experiments, such as those from telescopes or particle detectors, to extract meaningful information. |
Furthermore, the collaboration between physicists and data scientists is paving the way for innovative research methodologies. By leveraging machine learning, researchers can tackle complex problems that were previously deemed insurmountable. This interdisciplinary approach not only accelerates the pace of discovery but also fosters a richer understanding of the fundamental laws of the universe.
In essence, the integration of machine learning into physics is not just a trend; it's a revolution. As we continue to harness the power of artificial intelligence, the potential for groundbreaking discoveries expands exponentially. The future of physics is bright, and machine learning is at the forefront, illuminating paths that were once hidden in the shadows of uncertainty.
- What is machine learning? Machine learning is a subset of artificial intelligence that enables systems to learn from data and improve their performance without being explicitly programmed.
- How is machine learning used in physics? Machine learning is used for data analysis, predictive modeling, and anomaly detection, helping physicists to uncover new insights and enhance research efficiency.
- What are some examples of machine learning applications in physics? Examples include data classification in particle physics, predictive analytics in astrophysics, and image recognition in experimental setups.
- Why is machine learning important for future discoveries in physics? It allows researchers to process vast amounts of data quickly, identify patterns, and make predictions, accelerating the pace of scientific discovery.
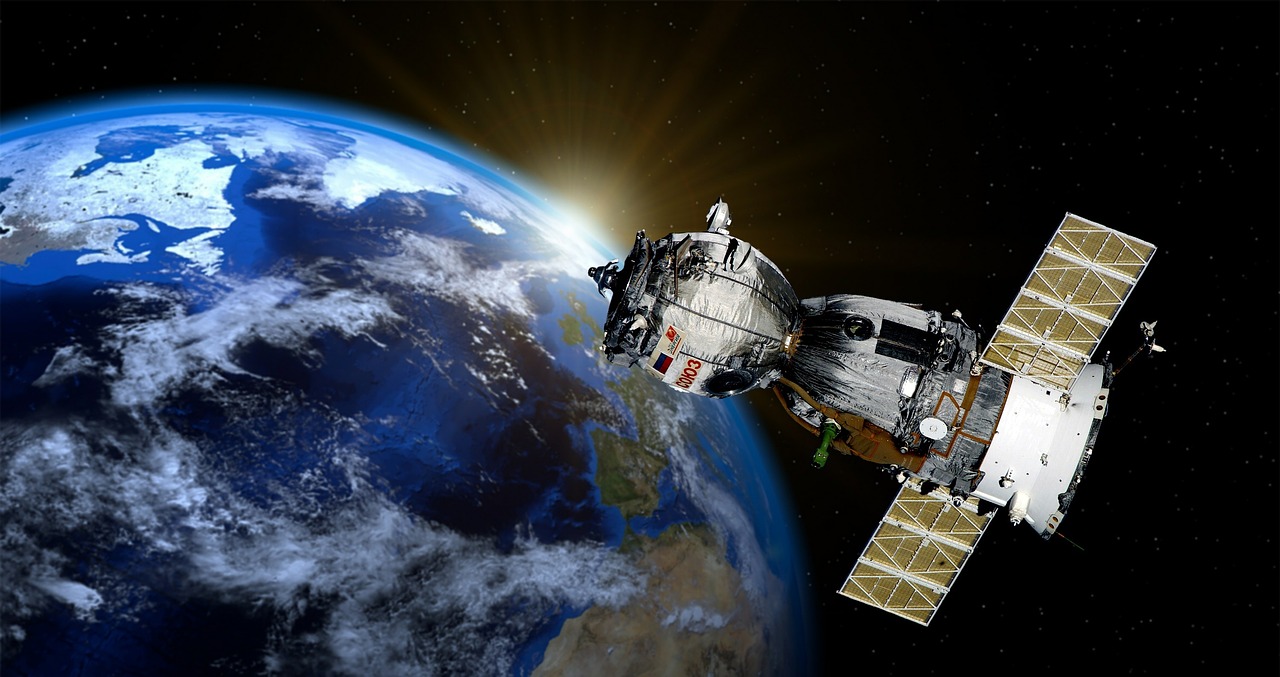
Data-Driven Discoveries
In the realm of physics, the integration of big data analytics has ushered in a new era of discovery, changing the way researchers approach their work. Imagine a vast ocean of data, swirling with potential insights just waiting to be uncovered. This ocean, once overwhelming, is now navigable thanks to advanced computational techniques that allow physicists to sift through and analyze enormous datasets with incredible speed and accuracy. The ability to process data at such unprecedented scales means that patterns which were once invisible can now be illuminated, leading to groundbreaking discoveries that redefine our understanding of the universe.
One of the most significant impacts of data-driven methodologies is the enhancement of experimental physics. Researchers are no longer confined to traditional methods of data collection and analysis. Instead, they can leverage sophisticated algorithms to identify correlations and anomalies within their datasets. For instance, in particle physics, experiments like those conducted at CERN generate petabytes of data daily. By employing machine learning techniques, scientists can filter through this data to spot rare events that may indicate new particles or interactions, potentially leading to revolutionary insights.
Moreover, the power of data-driven discoveries extends beyond particle physics. In fields such as astrophysics, astronomers utilize data from telescopes around the world to analyze cosmic events. Through the application of data mining techniques, they can uncover patterns in the light curves of distant stars, leading to the identification of exoplanets or understanding stellar evolution. The following table illustrates some key areas where data-driven discoveries are making waves:
Field | Application | Impact |
---|---|---|
Particle Physics | Event classification using machine learning | Discovery of new particles |
Astrophysics | Analysis of light curves | Identification of exoplanets |
Condensed Matter Physics | Data analysis for material properties | Development of new materials |
Furthermore, the collaborative nature of modern physics research means that data-driven discoveries often emerge from interdisciplinary teams. Physicists, computer scientists, and statisticians work side by side, merging their expertise to tackle complex problems. This synergy not only accelerates the pace of discovery but also fosters innovative approaches to long-standing questions in physics. As we continue to embrace the power of data, the potential for new breakthroughs seems limitless, paving the way for a deeper understanding of the fundamental laws that govern our universe.
- What is data-driven discovery in physics? Data-driven discovery refers to the use of advanced data analytics and computational techniques to uncover new insights and patterns in large datasets generated by experiments and observations in physics.
- How does machine learning contribute to physics research? Machine learning algorithms can analyze vast amounts of data quickly, helping physicists identify trends, classify events, and make predictions that may lead to new discoveries.
- What are some examples of successful data-driven discoveries? Examples include the identification of new particles at CERN, the discovery of exoplanets through light curve analysis in astrophysics, and advancements in material science through data analysis.
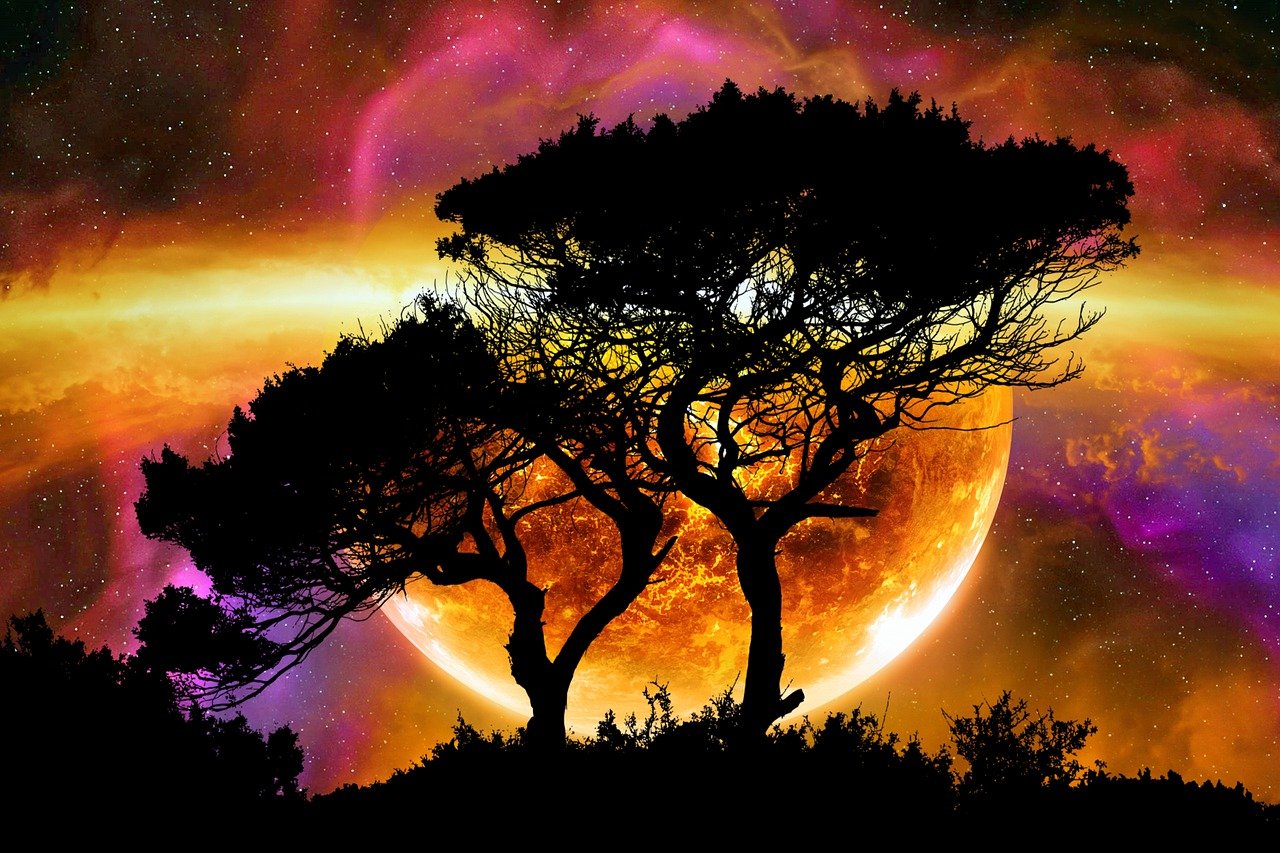
Modeling Astrophysical Phenomena
Astrophysics is a field that constantly pushes the boundaries of our understanding of the universe. With the advent of advanced modeling tools, physicists can now simulate a plethora of astrophysical events, from the birth of stars to the catastrophic explosions of supernovae. Imagine being able to visualize the merging of black holes or the dynamics of a galaxy forming over billions of years—all through the power of computation! These simulations not only help in visualizing complex phenomena but also in making predictions that can be tested against observational data.
One of the most exciting aspects of modeling astrophysical phenomena is the ability to recreate conditions that are otherwise impossible to observe directly. For instance, when simulating the conditions inside a neutron star, researchers can manipulate variables such as density and pressure to explore how matter behaves under extreme circumstances. The insights gained from these models are crucial for validating theoretical predictions and enhancing our understanding of the fundamental laws of physics.
To illustrate the impact of these models, consider the following table that highlights some key astrophysical phenomena and the modeling techniques used to study them:
Astrophysical Phenomenon | Modeling Technique | Key Insights |
---|---|---|
Black Hole Mergers | Numerical Relativity | Gravitational wave signals |
Galaxy Formation | Hydrodynamic Simulations | Structure and evolution of galaxies |
Supernova Explosions | Multi-Dimensional Simulations | Nucleosynthesis processes |
Cosmic Microwave Background | Cosmological Simulations | Understanding the early universe |
These models rely on complex algorithms and vast computational resources, often utilizing supercomputers to run simulations that can take weeks or even months to complete. The results can be breathtaking, providing stunning visualizations that are not only scientifically valuable but also captivating to the public. By bridging the gap between theoretical physics and observational astronomy, these simulations help us answer some of the most profound questions about the universe's origin, evolution, and ultimate fate.
Moreover, the models are not static; they evolve alongside advancements in technology and our understanding of physical laws. As new data from telescopes and space missions become available, astrophysicists can refine their models, making them more accurate and reliable. This iterative process is akin to a sculptor chiseling away at a block of marble, gradually revealing a masterpiece that reflects the intricate details of the cosmos.
In summary, modeling astrophysical phenomena is a dynamic and essential aspect of modern astrophysics. It empowers scientists to explore the universe's mysteries, offering a glimpse into the processes that govern everything from the smallest particles to the largest cosmic structures. The intersection of technology and physics in this domain not only enhances our knowledge but also ignites curiosity and wonder about the universe we inhabit.
- What are astrophysical phenomena? Astrophysical phenomena refer to events and processes that occur in space, such as star formation, black hole mergers, and supernova explosions.
- How do scientists model these phenomena? Scientists use advanced computational techniques and simulations to recreate the conditions of these events, allowing them to study their dynamics and effects.
- Why is modeling important in astrophysics? Modeling helps validate theoretical predictions, enhances our understanding of physical laws, and allows for the visualization of complex cosmic events.
- What tools are used for astrophysical modeling? Supercomputers, numerical relativity, and hydrodynamic simulations are commonly used tools in astrophysical modeling.
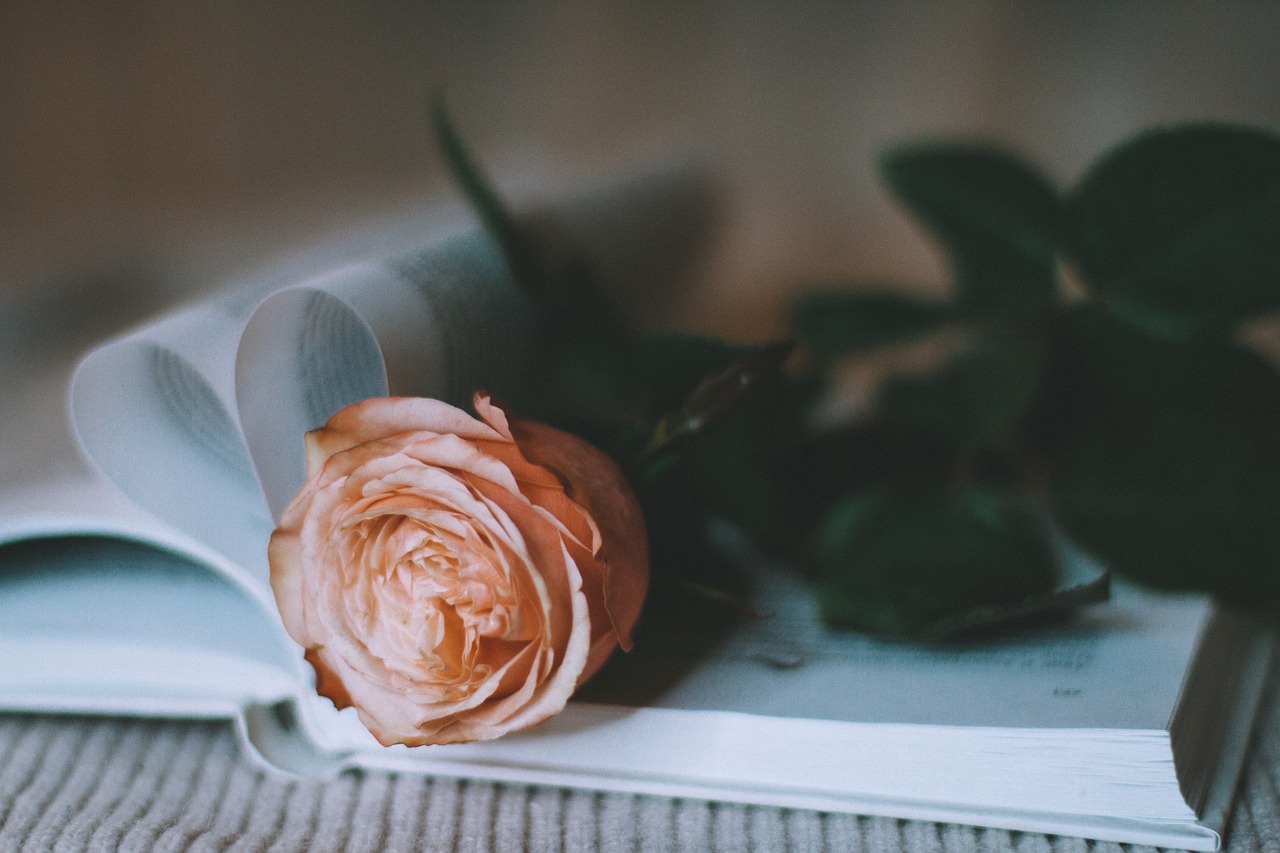
Impact on Education
The landscape of physics education is undergoing a **remarkable transformation** thanks to technology. Imagine a classroom where students can explore the laws of physics not just through textbooks, but through **interactive simulations** and **virtual labs** that bring complex concepts to life. This shift is not just about making learning more engaging; it's about making it more **accessible**. With the integration of technology, students from all walks of life can now delve into the fascinating world of physics, regardless of their geographical location or educational background.
One of the most significant changes is the rise of **online learning platforms**. These platforms have democratized access to quality education, allowing learners to study at their own pace. Whether it’s through video lectures, interactive quizzes, or discussion forums, students can engage with the material in a way that suits their learning style. This flexibility is a game-changer, especially for those who may have other commitments, like work or family. For instance, platforms like Coursera and edX offer courses from renowned universities, making it easier than ever to gain knowledge in physics.
Moreover, the advent of **virtual reality (VR)** technology is revolutionizing the way students experience physics. Imagine donning a VR headset and being transported into a 3D environment where you can observe the effects of gravitational forces or electromagnetic fields firsthand. This immersive experience not only makes learning more enjoyable but also enhances **retention** of complex ideas. Students can interact with simulations of physical phenomena, providing a hands-on approach that traditional classrooms often lack. This kind of learning is akin to stepping into a video game where the laws of physics govern every action, making it both fun and educational.
However, it’s not just about the tools available; it's also about how educators are adapting to these changes. Many instructors are now incorporating technology into their teaching methods, using tools like **interactive whiteboards** and **educational software** to create a more engaging classroom atmosphere. This shift encourages students to become active participants in their learning journey rather than passive recipients of information. The result? A generation of physicists who are not only knowledgeable but also **innovative thinkers** capable of tackling real-world challenges.
In summary, the impact of technology on physics education is profound and multifaceted. It’s creating a more inclusive, engaging, and effective learning environment that prepares students for the complexities of the modern world. As we continue to embrace these advancements, the future of physics education looks not just bright, but **limitless**.
- How has technology improved access to physics education?
Technology has provided online platforms and resources that allow students from various backgrounds to access quality education at their convenience. - What role does virtual reality play in learning physics?
Virtual reality offers immersive experiences that help students visualize and interact with complex physical concepts. - Are online courses as effective as traditional classroom settings?
Online courses can be equally effective, especially when they incorporate interactive elements and allow for self-paced learning. - How can educators integrate technology into their teaching?
Educators can use tools like interactive simulations, educational software, and online resources to enhance their teaching methods and engage students.
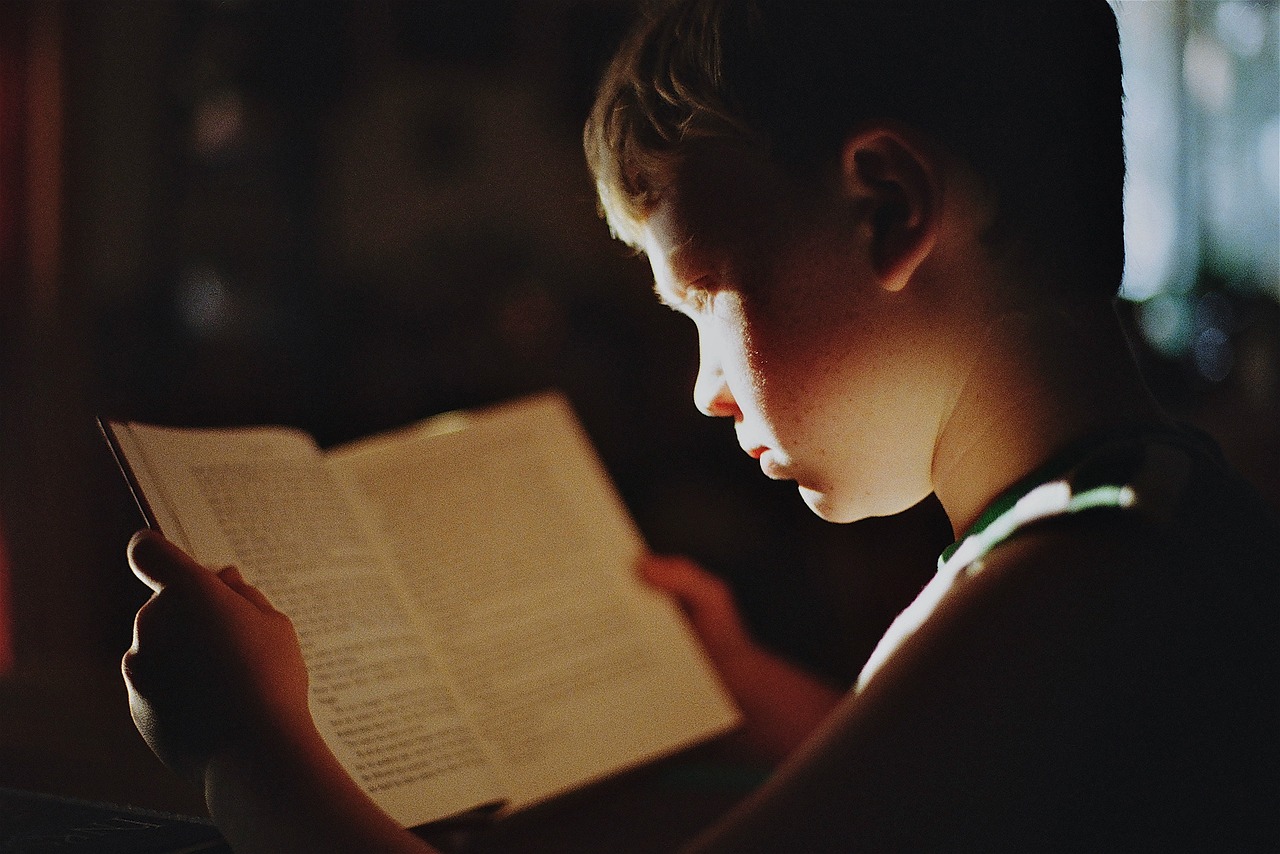
Online Learning Platforms
In today's fast-paced world, have emerged as a game-changer in the field of physics education. With the click of a button, students from all corners of the globe can access a treasure trove of resources that were once confined to the walls of traditional classrooms. Imagine being able to learn about the intricacies of quantum mechanics or the vastness of astrophysics without ever stepping foot in a lecture hall! These platforms not only provide flexibility but also cater to different learning styles, making physics more accessible than ever before.
One of the standout features of these platforms is the variety of courses available. From introductory classes to advanced topics, students can choose what suits their needs best. For instance, platforms like Coursera, edX, and Khan Academy offer a wide array of physics courses that cover everything from classical mechanics to modern theoretical physics. This democratization of knowledge means that anyone with an internet connection can dive into the mysteries of the universe.
Moreover, many of these platforms incorporate interactive elements such as quizzes, video lectures, and discussion forums. This engagement is crucial because it transforms passive learning into an active experience. Students can ask questions, participate in discussions, and even collaborate with peers from different backgrounds, enriching their understanding of complex concepts. The ability to learn at one's own pace is another significant advantage. Whether you are a night owl or an early bird, online platforms allow you to tailor your study schedule according to your personal rhythm.
Additionally, the use of technology in these platforms enhances the learning experience. For instance, some courses utilize simulation software that lets students visualize complex physical phenomena. Imagine being able to manipulate variables in a simulation of a particle collision or observing the effects of gravity on different celestial bodies in real-time! Such tools not only clarify theoretical concepts but also ignite a passion for discovery and inquiry among students.
As we continue to embrace this digital era, the future of physics education looks brighter than ever. With the constant evolution of technology, we can expect even more innovative features in online learning platforms, making physics not just a subject to study, but an exciting journey to embark on. The possibilities are endless, and the only limit is our curiosity!
- What are some popular online learning platforms for physics? Platforms like Coursera, edX, Khan Academy, and Udacity offer a wide range of physics courses.
- Can I learn physics at my own pace? Yes, most online platforms allow you to set your own learning schedule, making it easy to learn at your own pace.
- Are there interactive elements in online physics courses? Absolutely! Many courses include quizzes, simulations, and discussion forums to enhance the learning experience.
- Do I need prior knowledge to start learning physics online? While some courses are designed for beginners, others may require a foundational understanding of physics concepts.
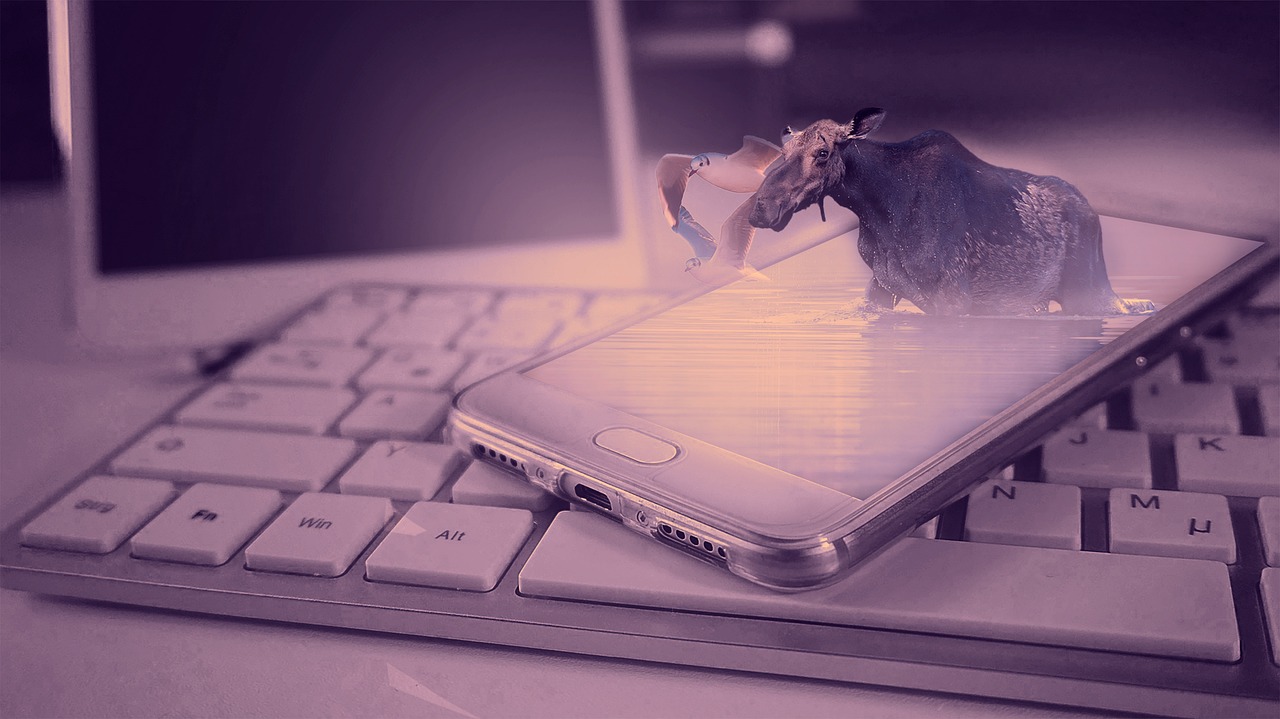
Virtual Reality Experiences
Imagine stepping into a world where complex physics concepts come to life right before your eyes. Virtual Reality (VR) is making this a reality, transforming the way students and enthusiasts engage with the subject. Rather than just reading about Newton's laws or electromagnetism, learners can now experience these principles in action. This immersive technology allows users to visualize intricate phenomena, making abstract ideas tangible and easier to grasp.
With VR, students can explore the solar system, witnessing the gravitational pull of planets, or simulate particle collisions in a controlled environment, all from the comfort of their classrooms. This hands-on approach fosters a deeper understanding of physics, as learners can manipulate variables and observe outcomes in real-time. For instance, by adjusting the angle of a projectile, students can see how it affects trajectory, reinforcing their grasp of motion principles.
Furthermore, VR experiences can cater to different learning styles. Visual learners benefit from seeing concepts illustrated in 3D, while kinesthetic learners thrive on interacting with digital objects. This adaptability to individual preferences is crucial in modern education, where one-size-fits-all approaches often fall short.
In addition to enhancing understanding, VR can also spark interest in physics among students who might otherwise find the subject daunting. By gamifying learning, students are more likely to engage with the material, leading to increased motivation and retention. Imagine a classroom where students are excited to explore the laws of thermodynamics through a VR game that challenges them to solve puzzles based on real-world physics scenarios!
However, the implementation of VR in physics education isn't without its challenges. The cost of VR equipment can be prohibitive for some institutions, and there is a learning curve associated with both the technology itself and the development of effective VR content. Nevertheless, as technology advances and becomes more accessible, the potential for VR to revolutionize physics education is immense.
In summary, Virtual Reality experiences are reshaping the landscape of physics education. By providing an interactive and immersive learning environment, VR not only enhances understanding but also inspires a new generation of physicists. As we continue to explore the possibilities of this technology, the future of physics education looks brighter than ever.
- What is Virtual Reality in education?
Virtual Reality (VR) in education refers to the use of immersive simulations that allow students to interact with and experience educational content in a 3D environment. - How does VR enhance learning in physics?
VR enhances learning by allowing students to visualize and manipulate complex physics concepts, making them more accessible and engaging. - Are VR experiences expensive to implement in schools?
Yes, the initial cost of VR equipment can be high, but prices are decreasing, and many schools are finding ways to integrate VR without breaking the bank. - Can VR be used for other subjects besides physics?
Absolutely! VR can be applied across various subjects, including biology, history, and mathematics, providing immersive experiences that enhance learning.
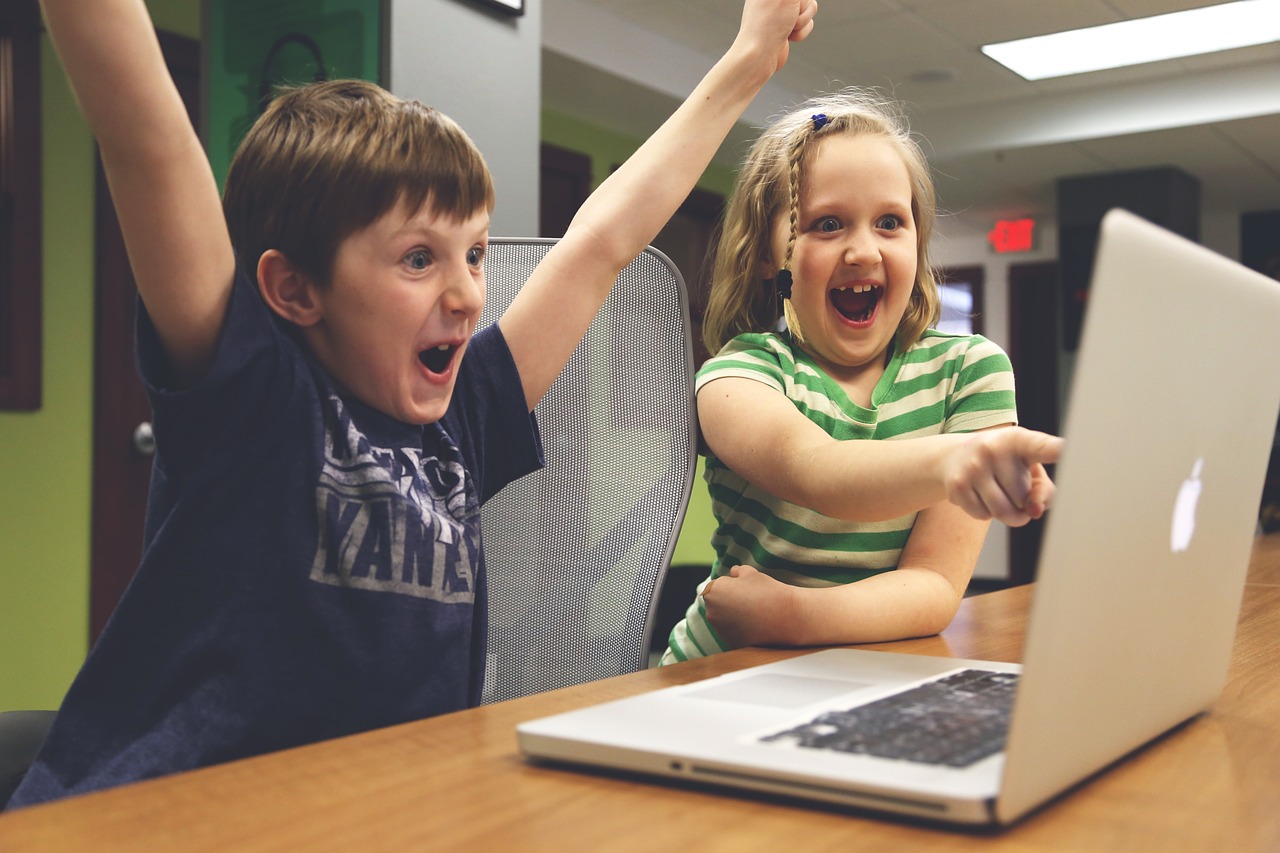
Interdisciplinary Collaborations
The world of physics is not a solitary endeavor; rather, it thrives on that push the boundaries of what we know. As technology continues to advance, the fusion of physics with other fields such as biology, engineering, and environmental science is becoming increasingly vital. This collaboration not only enhances the understanding of complex systems but also leads to innovative solutions that address real-world problems.
For instance, in the realm of biophysics, physicists are working hand-in-hand with biologists to unravel the mysteries of life at a molecular level. By utilizing advanced imaging technologies and analytical tools, researchers can observe biological processes in real-time, providing insights into how proteins fold, how cells communicate, and how diseases manifest. This synergy between disciplines is paving the way for breakthroughs in medical treatments and understanding human biology.
Similarly, in the field of environmental physics, the collaboration between physicists and environmental scientists is proving essential in tackling the pressing challenges posed by climate change. By employing sophisticated modeling techniques and data analytics, these teams can simulate environmental phenomena, predict climate patterns, and develop sustainable technologies. For example, the use of renewable energy sources is being optimized through physics-based simulations that assess efficiency and impact, leading to innovative solutions that are both effective and environmentally friendly.
Moreover, the intersection of physics with engineering has led to remarkable advancements in technology. Engineers utilize principles of physics to design everything from bridges to spacecraft, ensuring that structures are not only functional but also safe and efficient. The collaboration extends to the development of new materials, where physicists and engineers work together to create substances that can withstand extreme conditions or have unique properties, such as superconductivity.
As we look to the future, the importance of these interdisciplinary collaborations cannot be overstated. They are essential for driving innovation and addressing the complex challenges we face as a society. By combining expertise from various fields, researchers can tackle problems from multiple angles, leading to more comprehensive solutions that might not be possible within the confines of a single discipline.
In summary, the collaborative efforts between physics and other disciplines are not just enhancing our understanding of the universe; they are also creating a brighter future for humanity. As technology continues to evolve, the potential for groundbreaking discoveries and applications will only grow, making interdisciplinary collaboration an exciting frontier in the world of physics.
- What is interdisciplinary collaboration in physics?
Interdisciplinary collaboration in physics refers to the partnership between physicists and professionals from other fields, such as biology, engineering, and environmental science, to address complex problems and enhance research outcomes.
- How does technology facilitate these collaborations?
Technology provides advanced tools and platforms that allow researchers to share data, conduct simulations, and analyze results more efficiently, making it easier to work across disciplines.
- What are some examples of successful interdisciplinary projects?
Successful interdisciplinary projects include the development of medical imaging technologies in biophysics, the creation of sustainable energy solutions in environmental physics, and innovative material designs in engineering.
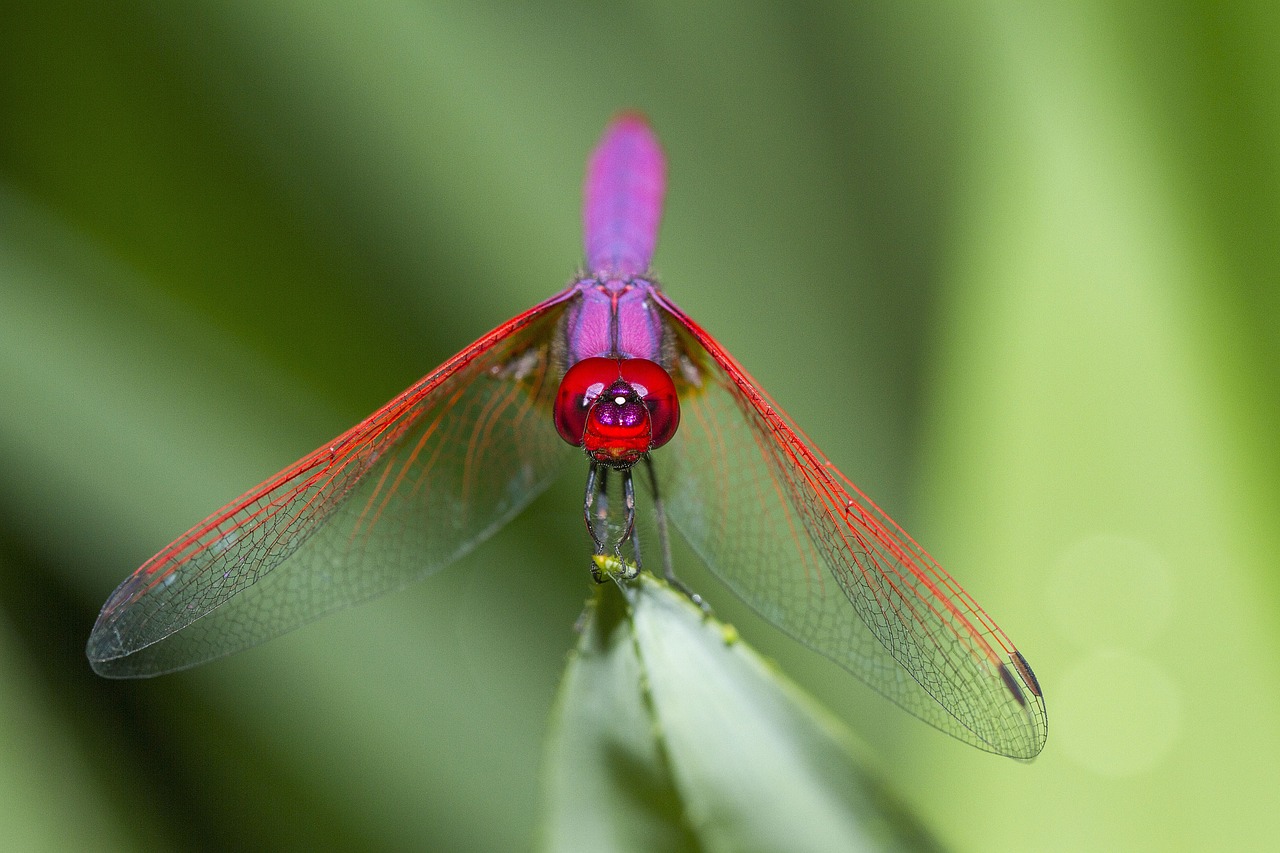
Biophysics Innovations
The field of biophysics is experiencing a remarkable transformation, driven by technological innovations that are reshaping our understanding of complex biological systems. Imagine being able to peer into the intricate workings of a cell or observe the interactions between proteins in real-time; this is now possible thanks to advanced imaging techniques and computational power. By harnessing tools such as fluorescence microscopy and electron microscopy, researchers can visualize biological processes at unprecedented resolutions, revealing details that were once hidden from view.
One of the most exciting advancements in biophysics is the development of single-molecule techniques. These methods allow scientists to study individual molecules rather than relying on averaged data from bulk samples. This shift is akin to going from watching a crowded concert to focusing on a single musician; it provides a clearer understanding of the dynamics at play. For instance, researchers can observe how enzymes function in real-time, offering insights into their mechanisms and efficiencies. Such detailed observations can lead to breakthroughs in drug design and disease treatment.
Furthermore, the integration of machine learning into biophysics is opening new avenues for discovery. By analyzing vast datasets generated from high-throughput experiments, machine learning algorithms can identify patterns and correlations that human researchers might overlook. This synergy between biology and technology is accelerating the pace of research, allowing scientists to make predictions about biological behavior and interactions that were previously unimaginable. For example, algorithms can predict protein folding, which is crucial for understanding diseases like Alzheimer's and cystic fibrosis.
Another significant innovation is the use of CRISPR technology in biophysics research. This revolutionary gene-editing tool not only allows for precise modifications of DNA but also facilitates the study of gene function and regulation. By creating models with specific genetic alterations, researchers can investigate the roles of individual genes in cellular processes, paving the way for targeted therapies and personalized medicine. The implications of this technology are profound, as it holds the potential to combat genetic disorders and improve health outcomes.
In addition to these advancements, the collaboration between biophysicists and engineers has led to the development of lab-on-a-chip technologies. These miniaturized devices integrate multiple laboratory functions on a single chip, enabling rapid analysis of biological samples. Imagine performing complex experiments in a fraction of the time and with significantly less material; this is the promise of lab-on-a-chip technology. It allows for real-time monitoring of biological processes, which is invaluable in fields such as drug testing and diagnostics.
Overall, the innovations in biophysics are not only enhancing our understanding of life at the molecular level but also driving practical applications that can transform healthcare and environmental sustainability. As technology continues to evolve, the potential for groundbreaking discoveries in biophysics is virtually limitless, offering a glimpse into the future of science where the boundaries between physics, biology, and technology blur.
- What is biophysics? Biophysics is an interdisciplinary field that applies the principles and methods of physics to understand biological systems.
- How has technology impacted biophysics? Technology has enabled advanced imaging techniques, machine learning applications, and innovative tools like CRISPR, significantly enhancing research capabilities.
- What are single-molecule techniques? Single-molecule techniques allow scientists to study individual molecules, providing detailed insights into their behavior and interactions.
- What is lab-on-a-chip technology? Lab-on-a-chip technology integrates multiple laboratory functions on a single chip, allowing for rapid and efficient analysis of biological samples.
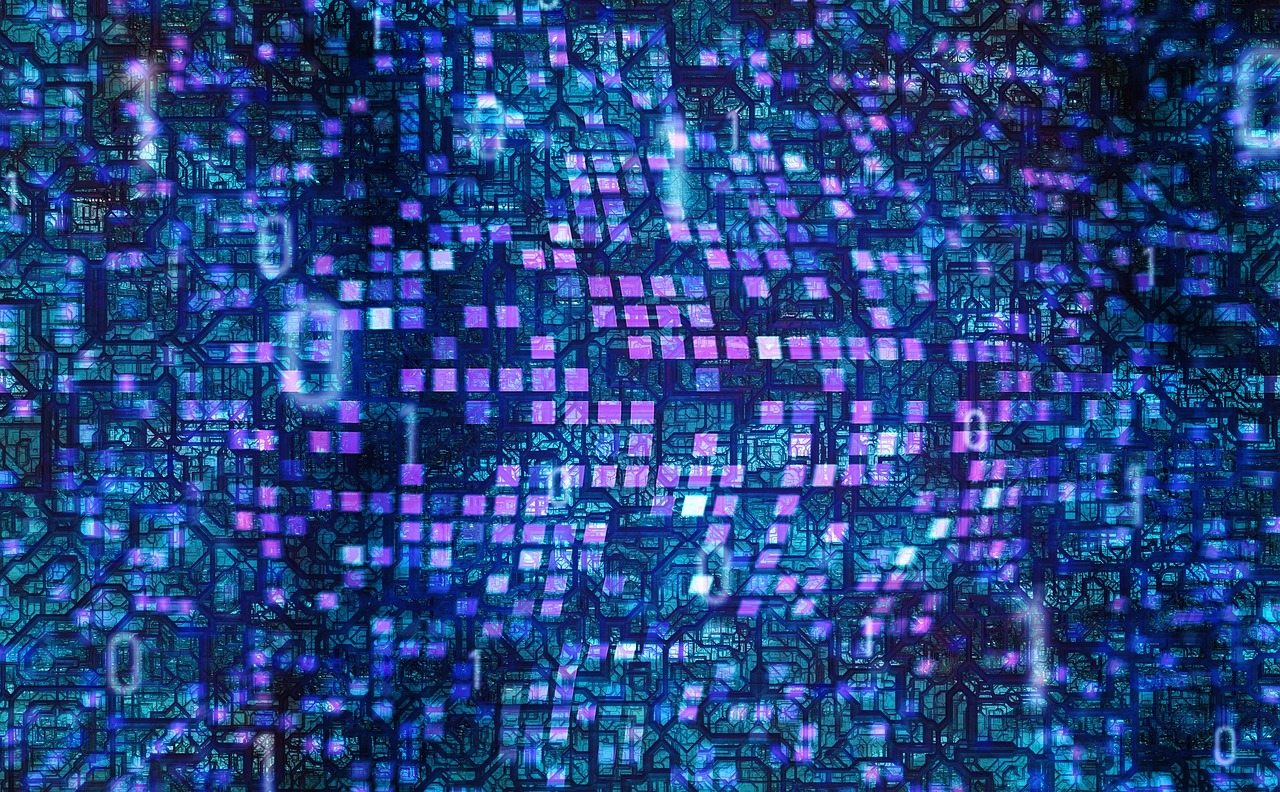
Environmental Physics
In today's world, where climate change and environmental degradation are pressing concerns, plays a crucial role in shaping our understanding and response to these challenges. By applying the principles of physics to environmental issues, scientists are developing innovative solutions that not only enhance our comprehension of natural phenomena but also pave the way for sustainable practices. Imagine a world where we can predict weather patterns with pinpoint accuracy or understand the intricate dynamics of ecosystems through the lens of physics. This is not just a dream; it's becoming a reality thanks to advancements in technology.
One of the most significant contributions of environmental physics is its ability to model complex systems. For instance, researchers use advanced computational tools to simulate the interactions between various environmental factors, such as temperature, humidity, and atmospheric pressure. These models help in predicting climate behavior and assessing the potential impacts of human activities on the environment. With these insights, policymakers can make informed decisions that promote sustainability and mitigate adverse effects.
Moreover, technology has enabled physicists to collect and analyze vast amounts of environmental data. This data-driven approach is crucial in understanding phenomena like global warming and air pollution. By employing sensors and satellites, scientists can monitor changes in the environment in real-time, allowing for quicker responses to emerging issues. For example, satellite imagery can track deforestation, while air quality sensors can provide immediate feedback on pollution levels in urban areas.
Additionally, the intersection of physics and environmental science has led to groundbreaking innovations in renewable energy. Technologies such as solar panels and wind turbines are rooted in physical principles, and ongoing research continues to enhance their efficiency. The development of smart grids—which optimize energy distribution based on real-time data—exemplifies how physics is applied to create sustainable energy solutions. These innovations not only reduce our reliance on fossil fuels but also contribute significantly to lowering greenhouse gas emissions.
As we delve deeper into the relationship between physics and the environment, it becomes clear that interdisciplinary collaboration is essential. Physicists often work alongside biologists, chemists, and engineers to tackle complex environmental problems. This collaborative spirit fosters innovative solutions that can address issues such as biodiversity loss and resource depletion. For instance, in the field of biophysics, researchers are exploring how physical principles can explain biological processes, leading to breakthroughs in conservation efforts.
In conclusion, environmental physics is a dynamic and evolving field that harnesses the power of technology to address some of the most pressing challenges of our time. By integrating physics with environmental science, we can develop sustainable practices that not only protect our planet but also enhance our quality of life. The future of environmental physics looks promising, as ongoing advancements continue to unveil new possibilities for understanding and preserving our world.
- What is environmental physics? Environmental physics is the study of physical principles and their application to environmental issues, including climate change, pollution, and renewable energy.
- How does technology influence environmental physics? Technology enhances data collection, modeling, and analysis, allowing for better predictions and solutions to environmental challenges.
- Why is interdisciplinary collaboration important in environmental physics? Collaborating with experts from various fields leads to innovative solutions that can effectively address complex environmental problems.
Frequently Asked Questions
- How has technology improved experimental physics?
Technology has dramatically enhanced experimental physics by providing tools that allow for more precise measurements and innovative techniques. This means scientists can now explore phenomena that were once considered unreachable, effectively pushing the boundaries of what we know about the physical world.
- What role does computational physics play in modern research?
Computational physics has become a cornerstone of theoretical research, enabling complex simulations and calculations. With powerful computing resources, physicists can delve deeper into the laws of the universe, uncovering insights that were previously impossible to achieve.
- What are quantum simulations, and why are they important?
Quantum simulations utilize the capabilities of quantum computing to model quantum systems. They provide insights into particle behavior and interactions that classical computers simply cannot replicate, making them crucial for advancing our understanding of fundamental physics.
- How is machine learning used in physics?
Machine learning techniques are increasingly applied in physics to analyze vast datasets. By uncovering patterns and making predictions in complex systems, machine learning aids researchers in making significant discoveries and enhancing their understanding of various phenomena.
- What impact does technology have on physics education?
Technology is transforming physics education by introducing interactive simulations and virtual labs, making complex concepts more accessible and engaging. Students can now visualize and experiment with physical principles in ways that were not possible before.
- How have online learning platforms changed physics education?
The rise of online learning platforms has democratized access to physics education, allowing learners around the globe to study at their own pace. This shift has opened doors for many who might not have had the opportunity to pursue physics otherwise.
- What are the benefits of virtual reality in learning physics?
Virtual reality technology offers immersive experiences that enable students to interact with physical concepts in a three-dimensional space. This hands-on approach enhances understanding and retention of complex ideas, making learning both fun and effective.
- How do interdisciplinary collaborations benefit physics?
Interdisciplinary collaborations foster innovative approaches in physics research, merging insights from fields like engineering and medicine. This synergy leads to breakthroughs that can address real-world challenges and expand our understanding of the universe.
- What advancements are being made in biophysics?
Technological advancements in imaging and analysis are driving breakthroughs in biophysics, helping researchers understand complex biological systems. This intersection of physics and biology is yielding exciting discoveries that could revolutionize medicine and health sciences.
- How is technology addressing environmental challenges in physics?
Technology plays a pivotal role in tackling environmental challenges by enabling physicists to develop sustainable solutions. Advanced modeling and data analysis techniques help researchers understand environmental systems and devise strategies to mitigate issues like climate change.