The Rise of Quantum Computing - What to Expect
The world is on the brink of a technological revolution, and at the center of this transformation is quantum computing. This cutting-edge field is not just a buzzword; it has the potential to redefine how we process information, solve problems, and even understand the universe. Imagine a computer that can perform calculations in seconds that would take classical computers thousands of years. Sounds like science fiction, right? But it's becoming a reality! As researchers and innovators dive deeper into the quantum realm, we are beginning to see a glimpse of the future—one where quantum computers could outperform their classical counterparts in numerous applications.
So, what exactly is quantum computing? At its core, it leverages the principles of quantum mechanics to process information in ways that classical computers simply cannot. While traditional computers use bits as the smallest unit of data, quantum computers use qubits, which can exist in multiple states simultaneously. This unique property allows quantum computers to tackle complex problems with unprecedented efficiency. It's akin to having a superpower in the world of computing, where the possibilities seem endless.
As we venture further into this exciting domain, it's crucial to understand the key technologies driving quantum computing. Technologies such as superconducting qubits, trapped ions, and quantum annealers are at the forefront of this innovation. Each of these technologies comes with its own set of advantages and challenges, contributing to the ongoing quest for practical applications. In the upcoming sections, we will explore these technologies in detail, highlighting their potential and the hurdles they face in becoming mainstream.
The implications of quantum computing are vast and varied. Industries ranging from technology to healthcare stand to benefit immensely. For instance, in the realm of cryptography, quantum computers could potentially break traditional encryption methods, leading to a significant shift towards quantum-resistant algorithms. In healthcare, the ability to simulate molecular interactions could drastically accelerate drug discovery, paving the way for more effective treatments tailored to individual patients.
However, it's not all smooth sailing. The path to widespread adoption of quantum computing is fraught with challenges. Technical hurdles such as maintaining qubit coherence and error correction are critical issues that need to be addressed. Additionally, the high costs associated with developing and maintaining quantum infrastructure pose significant barriers, especially for smaller organizations eager to explore this technology.
In conclusion, the rise of quantum computing is not just a technological advancement; it's a paradigm shift that could change the fabric of our society. As we stand on the cusp of this new era, it's essential to stay informed and engaged with the developments in this field. The future is quantum, and it promises to be nothing short of extraordinary.
- What is quantum computing? Quantum computing is a type of computation that utilizes quantum mechanics to process information in fundamentally different ways than classical computers.
- How are qubits different from bits? Unlike classical bits that can be either 0 or 1, qubits can exist in multiple states at once, allowing for greater computational power.
- What are some applications of quantum computing? Quantum computing has potential applications in cryptography, drug discovery, optimization problems, and more.
- What challenges does quantum computing face? Key challenges include technical hurdles, high costs, and the need for specialized expertise.
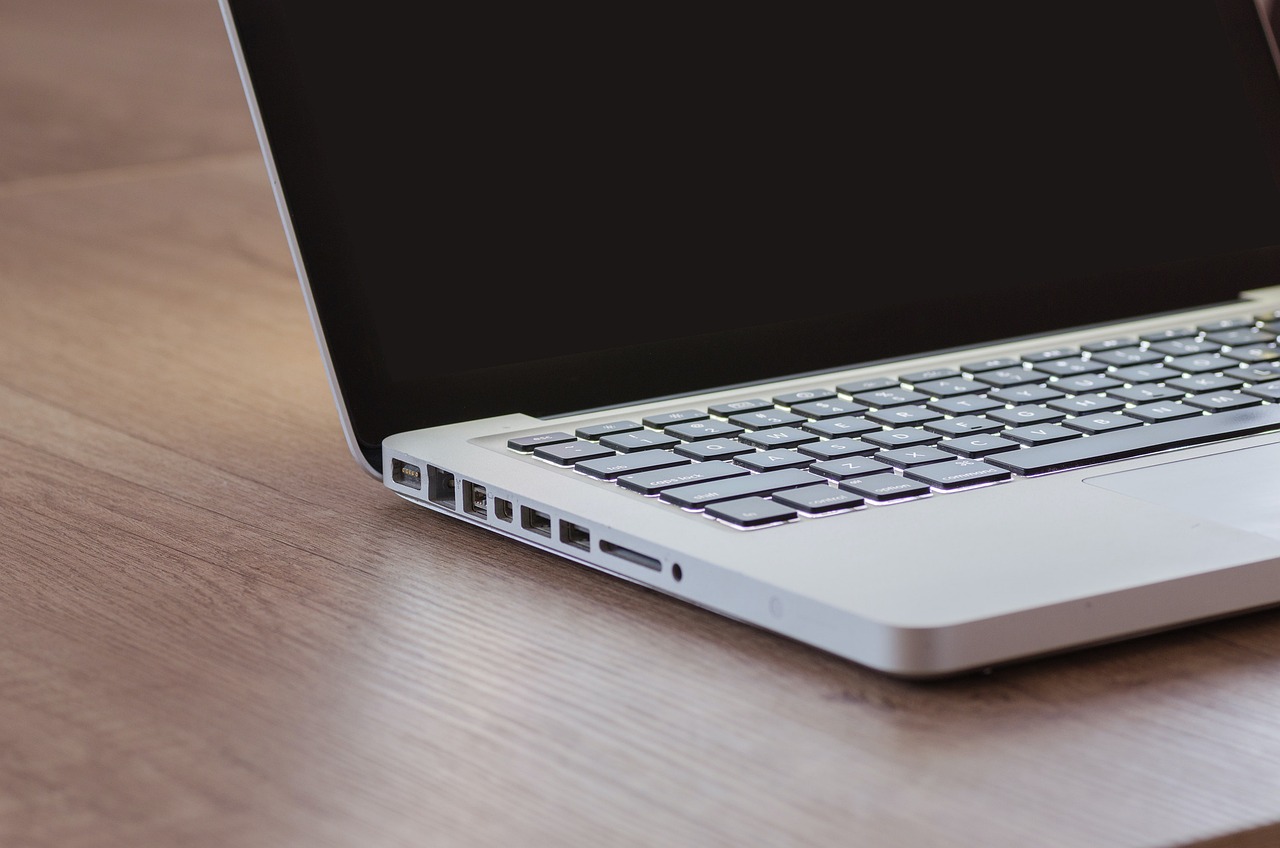
Understanding Quantum Computing
Quantum computing is a fascinating field that leverages the principles of quantum mechanics to process information in ways that are fundamentally different from classical computers. Imagine classical computers as very efficient librarians, quickly sorting through books to find the information you need. In contrast, quantum computers are like a magical library where every book is simultaneously open, allowing for an explosion of possibilities all at once. This unique capability stems from the use of qubits, the basic units of quantum information, which can exist in multiple states simultaneously due to a property known as superposition.
To put it simply, while classical bits can be either a 0 or a 1, qubits can be both at the same time, thanks to superposition. This characteristic allows quantum computers to perform complex calculations at speeds that would be unimaginable for traditional systems. Furthermore, qubits can also be entangled, meaning the state of one qubit is directly related to the state of another, no matter the distance between them. This entanglement creates a powerful network of information that can be manipulated in ways that classical computers simply cannot replicate.
The implications of quantum computing are enormous. From solving intricate mathematical problems to optimizing logistics in real-time, quantum computers promise to tackle challenges that are currently beyond our reach. For instance, they could revolutionize fields such as cryptography, where they can crack encryption codes that protect sensitive data, or in healthcare, where they can simulate complex biological processes to accelerate drug discovery.
However, the journey towards practical quantum computing is not without its challenges. Maintaining the delicate state of qubits requires extremely low temperatures and sophisticated error correction methods, which add layers of complexity to the technology. Researchers are tirelessly working to overcome these hurdles, aiming to unlock the full potential of quantum computing and bring it into the mainstream.
In summary, quantum computing stands at the forefront of technological innovation, promising to transform our approach to problem-solving across various sectors. As we continue to explore this intriguing realm, the question remains: how quickly will we see these advancements translate into real-world applications? The future holds exciting possibilities, and it’s a journey worth following.
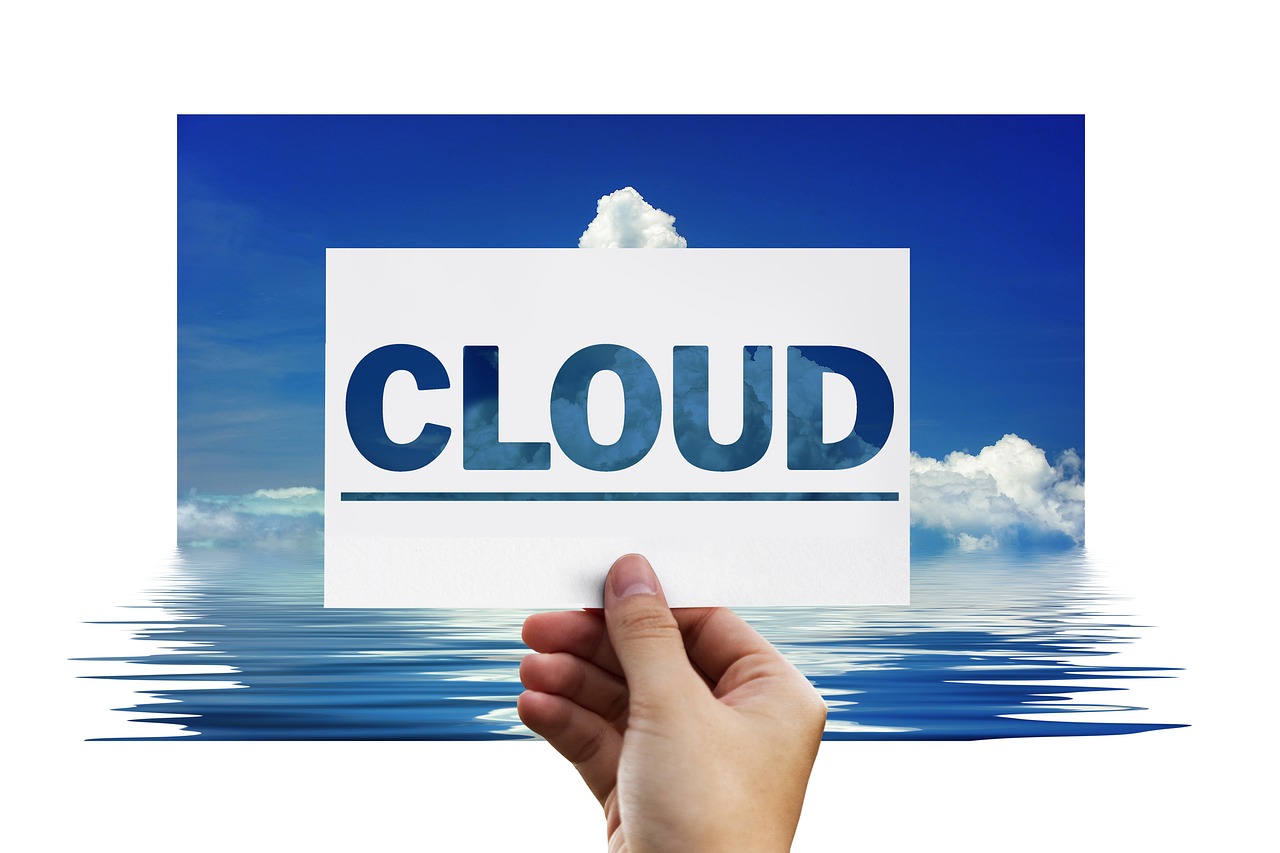
Key Technologies Driving Quantum Computing
As we delve into the realm of quantum computing, it becomes essential to understand the key technologies that are propelling this revolutionary field forward. At the heart of quantum computing are several groundbreaking technologies, each bringing unique characteristics and challenges to the table. These include superconducting qubits, trapped ions, and quantum annealers. Each of these technologies operates on the principles of quantum mechanics but does so in distinct ways that influence their performance and applicability.
Firstly, superconducting qubits have emerged as one of the most promising avenues in quantum computing. They utilize superconducting materials to create qubits that can be manipulated at incredibly high speeds. This rapid manipulation allows for the construction of larger quantum systems, which is crucial for tackling complex computational challenges. However, it's important to note that while they offer remarkable advantages in speed and integration, they also grapple with issues related to coherence times and error rates, which can undermine their reliability.
When we talk about superconducting qubits, we must highlight their significant advantages. These qubits are not only fast but also highly integrable, making them ideal for scaling up quantum systems. Their ability to perform multiple operations in parallel is akin to having a highly efficient factory where many products are being assembled simultaneously. This characteristic positions superconducting qubits as a leading contender in the race toward practical quantum computing.
The advantages of superconducting qubits can be summarized as follows:
- High Speed: They allow for rapid qubit manipulation, which is essential for efficient computation.
- Scalability: Their design facilitates the construction of larger quantum circuits.
- Integration: They can be integrated with existing semiconductor technologies, enhancing their accessibility.
Despite their promise, superconducting qubits face significant challenges that must be addressed. The primary challenges include:
- Coherence Times: Maintaining the state of qubits for extended periods is critical, yet difficult.
- Error Rates: High error rates can lead to inaccurate computations, necessitating robust error correction methods.
Another pivotal technology is trapped ions. This method employs individual ions that are suspended in electromagnetic fields and manipulated using laser beams. The precision offered by trapped ions is remarkable, allowing for highly stable qubit operations. However, the scalability of this technology remains a significant challenge, as managing a large number of ions becomes increasingly complex. Think of it like trying to organize a large orchestra; while each musician can play beautifully, coordinating them all to perform in harmony is no small feat!
In summary, the key technologies driving quantum computing—superconducting qubits, trapped ions, and quantum annealers—each present unique advantages and hurdles. As researchers continue to explore these technologies, the potential for groundbreaking advancements in quantum computing becomes ever more tangible.
- What is quantum computing?
- Quantum computing is a type of computation that uses quantum mechanics to process information in ways that classical computers cannot, enabling solutions to complex problems.
- Why are superconducting qubits important?
- Superconducting qubits are crucial because they allow for faster computations and can be scaled up to create larger quantum systems, making them a leading technology in quantum computing.
- What challenges do trapped ions face?
- Trapped ions offer high precision but struggle with scalability, making it challenging to manage and manipulate a large number of qubits effectively.
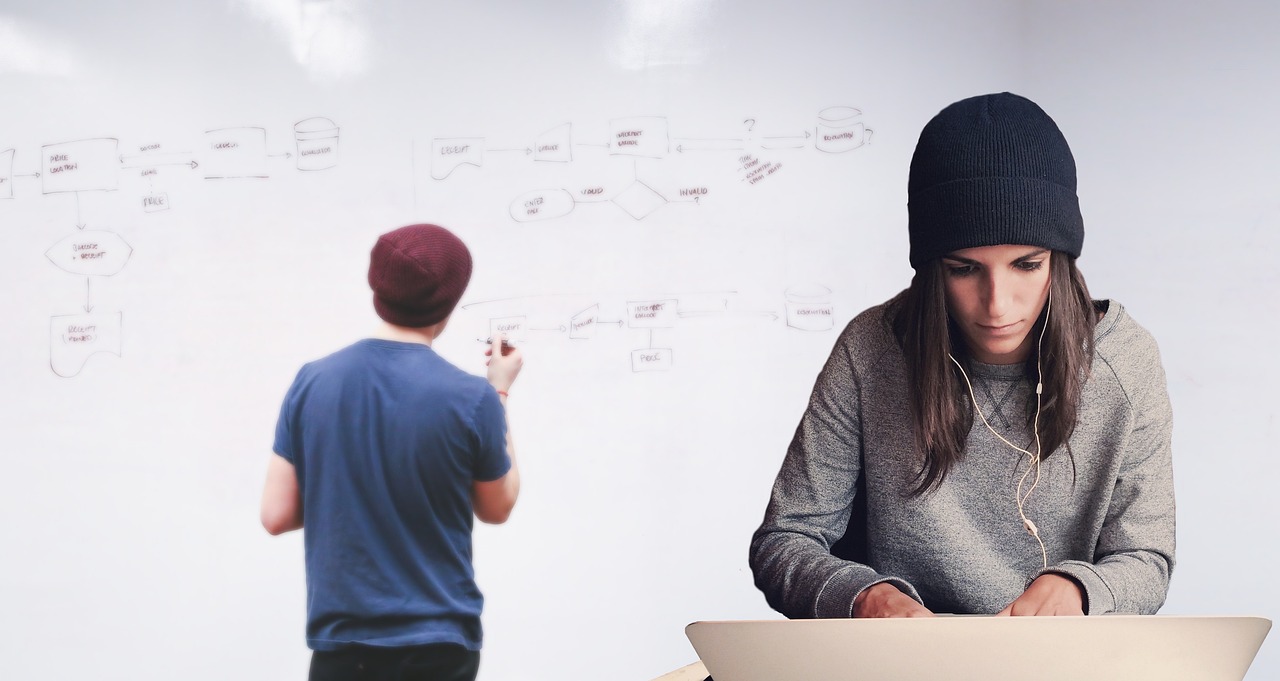
Superconducting Qubits
Superconducting qubits are rapidly becoming the **rock stars** of the quantum computing world. They are essentially tiny circuits made from superconducting materials that can carry an electric current without resistance when cooled to extremely low temperatures. This unique property allows them to operate with incredible speed and efficiency, making them a prime candidate for building powerful quantum computers. Imagine a supercharged race car zooming around a track—this is what superconducting qubits can do for computational tasks that would take classical computers eons to solve.
One of the standout features of superconducting qubits is their ability to be manipulated with high precision. Researchers can perform operations on these qubits in mere nanoseconds, allowing for rapid processing of quantum information. This speed is crucial, especially when you consider the complex calculations and simulations that quantum computers are designed to tackle. However, it's not all smooth sailing; despite their advantages, superconducting qubits face significant challenges, particularly when it comes to maintaining coherence—the ability to keep the qubit in a stable quantum state long enough to perform calculations.
To illustrate the advantages and challenges of superconducting qubits, we can take a closer look at their characteristics in the following table:
Advantages | Challenges |
---|---|
|
|
In summary, superconducting qubits are a **double-edged sword**—they offer tremendous potential and speed but come with their own set of challenges that researchers are actively working to overcome. The field is evolving rapidly, and as advancements continue to be made, we can expect superconducting qubits to play a pivotal role in the future of quantum computing. With ongoing innovations, who knows? We might soon witness breakthroughs that were once thought to be the stuff of science fiction.
- What are superconducting qubits? Superconducting qubits are circuits made from superconducting materials that can perform quantum computations with high speed and efficiency.
- What challenges do superconducting qubits face? They struggle with maintaining coherence and managing error rates, which are critical for reliable quantum computations.
- Why are superconducting qubits important? They are key to building scalable quantum systems that can solve complex problems beyond the reach of classical computers.
- How do superconducting qubits compare to other types of qubits? They offer high speed and integration potential but face challenges related to temperature and coherence compared to other technologies like trapped ions.
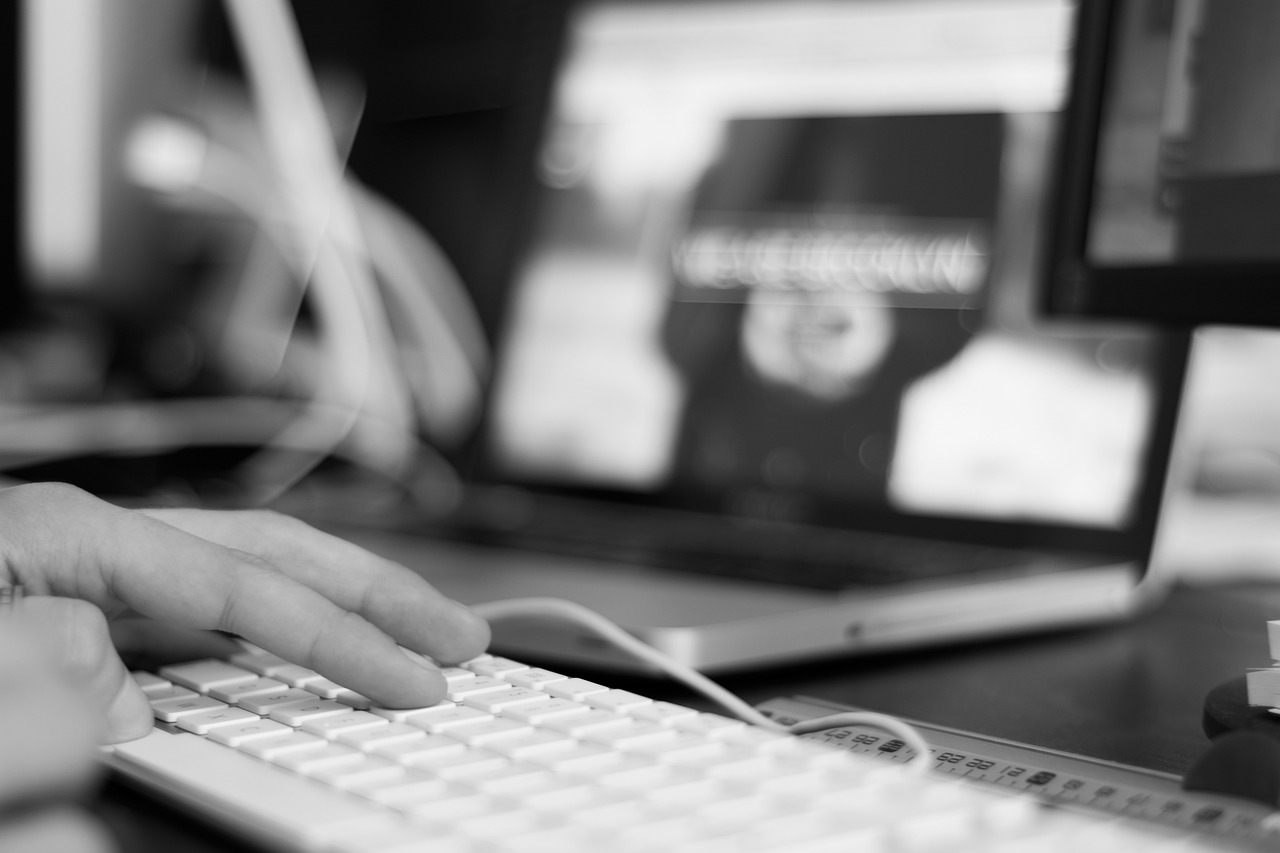
Advantages of Superconducting Qubits
Superconducting qubits stand out as one of the most promising technologies in the quantum computing landscape. They offer a unique blend of speed and integration capabilities that make them particularly suitable for building larger quantum systems. Imagine being able to tackle computational problems that would take classical computers centuries to solve—this is the potential that superconducting qubits bring to the table.
One of the key advantages of superconducting qubits is their ability to perform rapid qubit manipulation. This speed is crucial when you consider the complex algorithms that quantum computers utilize. With superconducting qubits, researchers can execute operations in mere microseconds, allowing for more complex computations to be performed in a shorter time frame. This rapid processing capability is akin to having a sports car on a racetrack, where every second counts.
Moreover, the integration capabilities of superconducting qubits allow for the construction of larger quantum circuits. This is essential because, in quantum computing, the more qubits you have, the more powerful your system becomes. By combining multiple superconducting qubits, scientists can create intricate quantum circuits that can solve complex problems more efficiently. For instance, consider a massive jigsaw puzzle; the more pieces you have, the clearer the picture becomes. Similarly, more qubits lead to more powerful computational abilities.
However, it’s not just about speed and integration; superconducting qubits also benefit from advancements in fabrication techniques. These advancements have made it possible to produce qubits with greater precision and reliability. As a result, superconducting qubits are becoming increasingly viable for practical applications, ranging from optimization problems to machine learning. This is where the real magic happens—when theoretical capabilities translate into real-world applications that can significantly impact various industries.
In summary, the advantages of superconducting qubits lie in their:
- High Speed: Enabling rapid qubit manipulation for complex computations.
- Integration Capabilities: Facilitating the construction of larger quantum systems.
- Advanced Fabrication Techniques: Leading to greater precision and reliability.
As we look to the future, the combination of these advantages positions superconducting qubits as a key player in the quest for practical quantum computing solutions. With ongoing research and development, we can expect to see even more innovative applications that harness the unique properties of these qubits, pushing the boundaries of what we thought was possible.
Q1: What are superconducting qubits?
A1: Superconducting qubits are a type of quantum bit that uses superconducting materials to perform quantum computations. They are known for their high speed and integration capabilities.
Q2: What are the main advantages of using superconducting qubits?
A2: The main advantages include rapid qubit manipulation, the ability to build larger quantum systems, and advancements in fabrication techniques that enhance their precision and reliability.
Q3: How do superconducting qubits compare to other types of qubits?
A3: While superconducting qubits excel in speed and scalability, other types like trapped ions may offer better coherence times and stability. Each technology has its own set of advantages and challenges.
Q4: What industries can benefit from superconducting qubits?
A4: Industries such as finance, healthcare, and logistics can benefit from superconducting qubits, particularly in areas like optimization problems, drug discovery, and complex simulations.
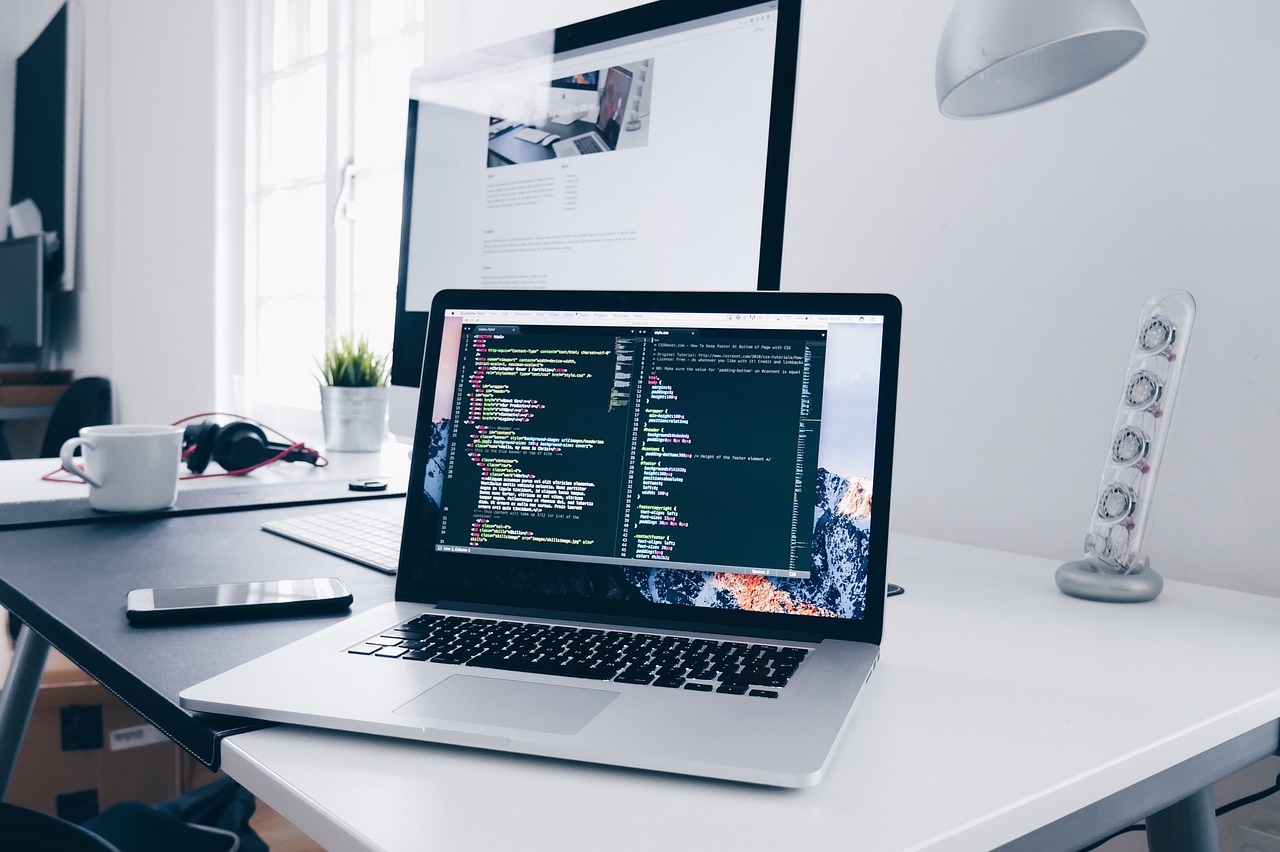
Challenges of Superconducting Qubits
While superconducting qubits are heralded as a leading technology in the quantum computing landscape, they are not without their significant challenges. One of the primary issues is maintaining coherence, which refers to the qubit's ability to maintain its quantum state over time. In simpler terms, coherence is like a tightrope walk; if the qubit loses its balance, the delicate quantum information can easily fall into chaos, leading to errors in computation.
Moreover, the error rates associated with superconducting qubits present another hurdle. These errors can arise from various sources, including environmental noise and imperfections in the qubit's design. To illustrate, think of a game of telephone where a message is passed along a line of people. If even one person mishears the message, the final outcome can be drastically different from the original. In quantum computing, this miscommunication can result in faulty calculations, which is a significant concern for researchers aiming for reliable quantum systems.
To combat these challenges, researchers are exploring various solutions, including:
- Error correction codes that can identify and correct errors in real-time.
- Improved materials that enhance qubit stability and coherence times.
- Advanced cooling techniques to minimize thermal noise that disrupts qubit operations.
Despite these efforts, achieving a practical and reliable superconducting qubit system remains a work in progress. The ongoing research is crucial, as the potential benefits of overcoming these challenges could lead to breakthroughs in quantum computing that would transform industries from finance to healthcare. As we advance, the quest for better coherence and lower error rates will be at the forefront of quantum technology development.
- What are superconducting qubits? Superconducting qubits are a type of quantum bit that utilizes superconducting materials to perform quantum computations.
- Why is coherence important in quantum computing? Coherence is essential as it allows qubits to maintain their quantum state, which is crucial for accurate calculations.
- What are the main challenges faced by superconducting qubits? The main challenges include maintaining coherence, managing error rates, and ensuring scalability for practical applications.
- How are researchers addressing these challenges? Researchers are developing error correction codes, improving materials, and exploring advanced cooling techniques to enhance qubit performance.

Trapped Ions
Trapped ion technology stands as a beacon of precision and stability in the realm of quantum computing. Utilizing individual ions that are held in place by electromagnetic fields, this method allows researchers to manipulate quantum bits (qubits) with incredible accuracy using lasers. The beauty of trapped ions lies in their ability to maintain quantum states for extended periods, which is crucial for performing complex calculations. Imagine trying to balance a spinning top on your finger; the longer you can keep it spinning, the more tricks you can perform. Similarly, the stability of trapped ions enables more intricate quantum operations.
However, despite these advantages, scalability remains a significant hurdle. While a handful of trapped ions can be controlled with high precision, scaling up to a system that can outperform classical computers requires managing many more ions simultaneously. This challenge is akin to conducting a symphony orchestra—while a few musicians can play beautifully together, adding more instruments complicates the harmony and coordination. Researchers are actively exploring various methods to enhance scalability, including novel laser techniques and innovative ion trap designs.
In terms of practical applications, trapped ion quantum computers have already demonstrated their potential in areas such as quantum simulation and error correction. They excel in tasks that require high fidelity and low error rates, making them suitable for foundational research in quantum algorithms. Nevertheless, the journey to widespread adoption is paved with obstacles. The intricate setup and maintenance of trapped ion systems demand specialized expertise and significant investment, which can deter smaller organizations from entering the quantum arena.
As we look to the future, the advancements in trapped ion technology could lead to breakthroughs that redefine our understanding of computation. Companies and research institutions are pouring resources into this field, driven by the prospect of solving problems that are currently deemed impossible for classical systems. The race is on, and while the finish line may still be distant, the excitement surrounding trapped ions is palpable.
- What are trapped ions? Trapped ions are charged atoms that are confined using electromagnetic fields, allowing for precise control and manipulation in quantum computing.
- How do trapped ions work in quantum computing? Trapped ions use lasers to manipulate the quantum states of individual ions, enabling complex calculations and operations necessary for quantum computing.
- What are the advantages of using trapped ions? Trapped ions offer high precision, long coherence times, and low error rates, making them suitable for foundational quantum research.
- What challenges do trapped ions face? The primary challenges include scalability, high costs, and the need for specialized expertise to maintain and operate the systems.
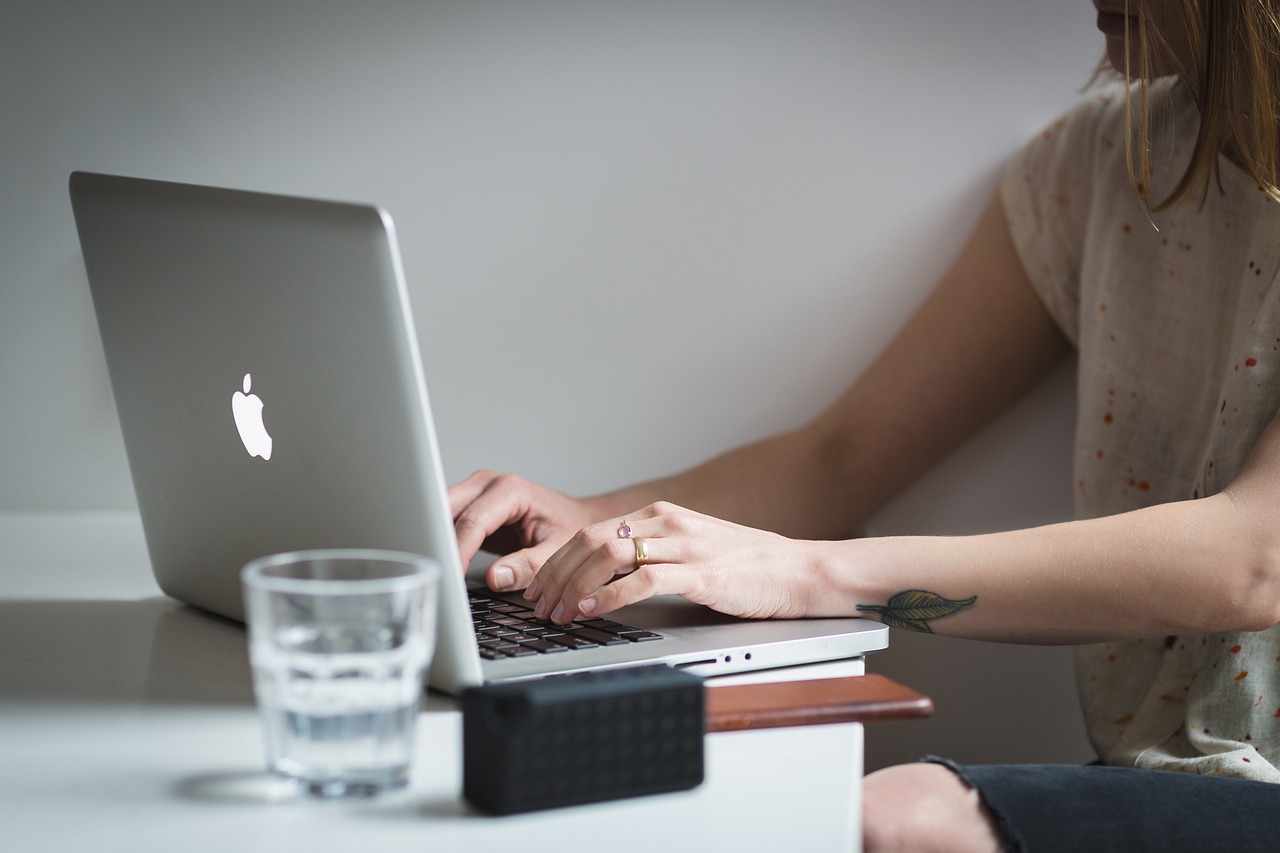
Applications of Quantum Computing
Quantum computing is not just a futuristic concept; it is poised to revolutionize various fields by tackling problems that are currently beyond the reach of classical computers. Imagine a world where complex calculations that take years can be done in mere seconds! This is the promise of quantum computing. Its applications span across several sectors, including cryptography, drug discovery, and optimization problems. Each of these areas stands to benefit immensely from the unparalleled computational power that quantum computers offer.
One of the most exciting implications of quantum computing is its impact on cryptography. Traditional encryption methods, which have long been the backbone of digital security, could be rendered obsolete. Quantum computers possess the capability to break these encryption schemes with relative ease, leading to a pressing need for the development of quantum-resistant algorithms. This shift is crucial for safeguarding sensitive information in an increasingly digital world. As we transition into this new era, organizations must adapt to stay ahead of potential threats.
In the realm of healthcare, quantum computing holds the potential to accelerate drug discovery significantly. By simulating molecular interactions at an unprecedented scale, researchers can identify promising drug candidates much faster than traditional methods allow. This capability could lead to breakthroughs in treatments for various diseases, enabling the development of personalized medicine approaches tailored to individual patients. The speed at which quantum computing can process complex biological data could transform how we understand and treat illnesses, ultimately saving lives and reducing healthcare costs.
Moreover, quantum computing can also optimize complex systems in ways that classical computers struggle to achieve. For example, in logistics and supply chain management, quantum algorithms can analyze vast amounts of data to find the most efficient routes and methods for transporting goods. This optimization can lead to significant cost savings and reduced environmental impact, making businesses not only more profitable but also more sustainable.
As we explore these applications, it's essential to consider the potential challenges and limitations that come with them. While the promise of quantum computing is immense, the transition from theory to practical application requires overcoming significant hurdles. Nonetheless, the possibilities are exciting, and as advancements continue, we can expect to see quantum computing play a pivotal role in shaping the future.
- What is quantum computing?
Quantum computing leverages the principles of quantum mechanics to process information in ways that classical computers cannot, enabling faster and more efficient problem-solving. - How does quantum computing impact cryptography?
Quantum computers can break traditional encryption methods, necessitating the development of quantum-resistant algorithms to protect sensitive data. - Can quantum computing accelerate drug discovery?
Yes, by simulating molecular interactions quickly, quantum computing can significantly speed up the drug discovery process, leading to more effective treatments. - What are the main challenges facing quantum computing?
Key challenges include technical hurdles, high costs, and the need for specialized expertise, which can delay widespread adoption.
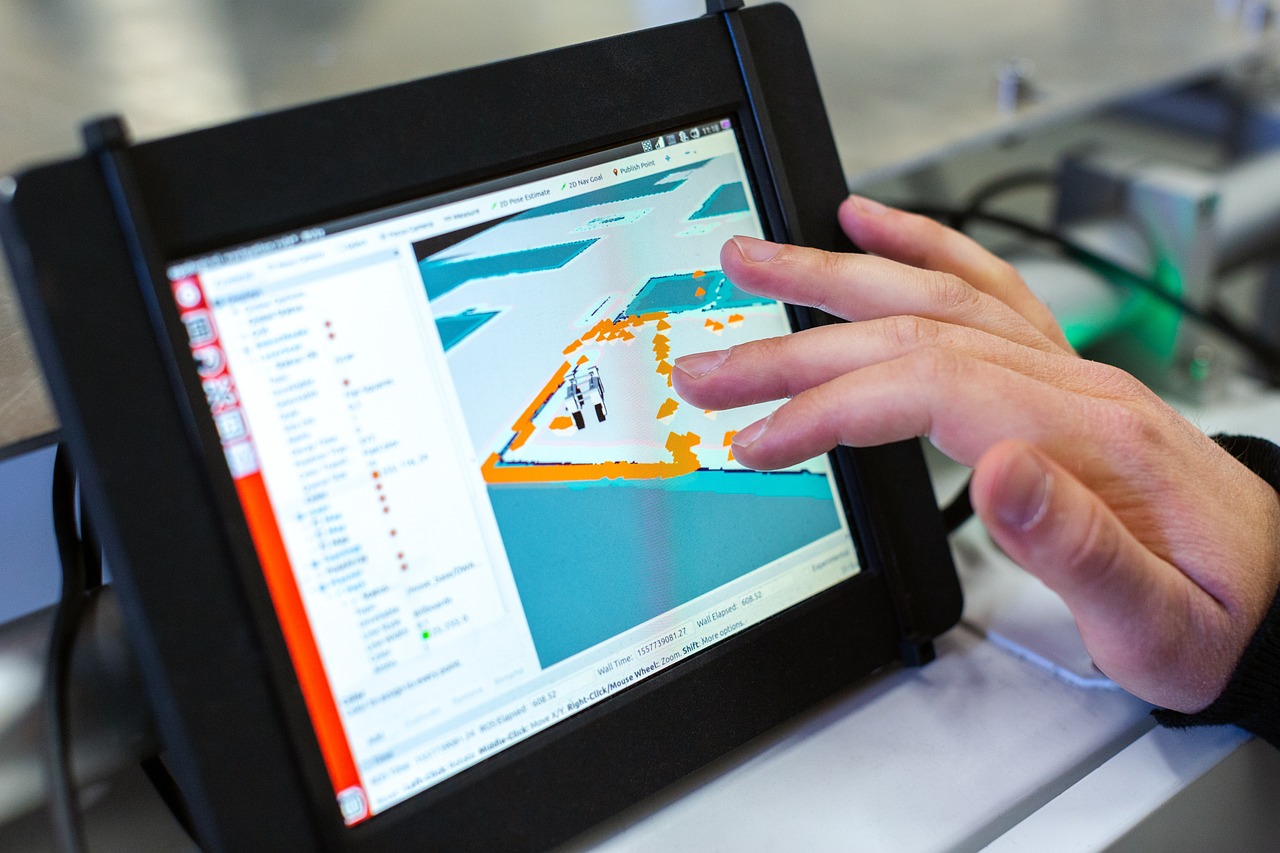
Impact on Cryptography
As we stand on the brink of a technological revolution, the impact of quantum computing on cryptography cannot be overstated. Traditional encryption methods, which have served as the backbone of digital security for decades, are now facing an existential threat. Imagine a world where sensitive information, from personal data to national secrets, could be easily deciphered by a quantum computer. This scenario isn't just a plot from a sci-fi movie; it's a real possibility that has experts around the globe scrambling to develop quantum-resistant algorithms.
Current encryption techniques, such as RSA and ECC (Elliptic Curve Cryptography), rely on the difficulty of certain mathematical problems. For instance, RSA’s security is based on the challenge of factoring large prime numbers. However, quantum computers, with their ability to perform complex calculations at unprecedented speeds, could crack these codes in a fraction of the time it takes classical computers. The famous Shor’s algorithm illustrates this capability, demonstrating that a sufficiently powerful quantum computer could factor large numbers exponentially faster than its classical counterpart.
To mitigate these risks, the cybersecurity community is actively researching and implementing new cryptographic methods that can withstand quantum attacks. These methods fall under the umbrella of post-quantum cryptography and focus on algorithms that are resistant to quantum decryption techniques. Some promising candidates include:
- Lattice-based cryptography: This relies on the hardness of lattice problems, which are believed to be difficult even for quantum computers.
- Hash-based cryptography: Utilizing hash functions to create secure signatures that are not easily broken by quantum algorithms.
- Multivariate polynomial cryptography: Based on the difficulty of solving systems of multivariate polynomial equations.
Transitioning to these new cryptographic standards is not just a technical challenge; it’s a race against time. As quantum computing technology continues to advance, the urgency to implement these new systems becomes paramount. Governments, corporations, and individuals must prepare for a future where their data's security depends on the resilience of their cryptographic methods against quantum threats.
In conclusion, the impact of quantum computing on cryptography is profound and far-reaching. It necessitates a shift in how we think about data security and privacy. The race is on to develop quantum-resistant algorithms that can ensure the integrity of our digital lives. While the challenges are significant, the rewards for successfully navigating this transition could be monumental, paving the way for a new era of cybersecurity.
- What is quantum computing? Quantum computing utilizes the principles of quantum mechanics to process information in ways that classical computers cannot, enabling faster and more complex computations.
- How does quantum computing threaten current encryption methods? Quantum computers can solve certain mathematical problems, such as factoring large numbers, much faster than classical computers, potentially breaking traditional encryption methods.
- What is post-quantum cryptography? Post-quantum cryptography refers to cryptographic algorithms that are believed to be secure against the capabilities of quantum computers.
- How can I protect my data from quantum threats? Transitioning to quantum-resistant algorithms and staying informed about developments in cybersecurity can help protect your data.
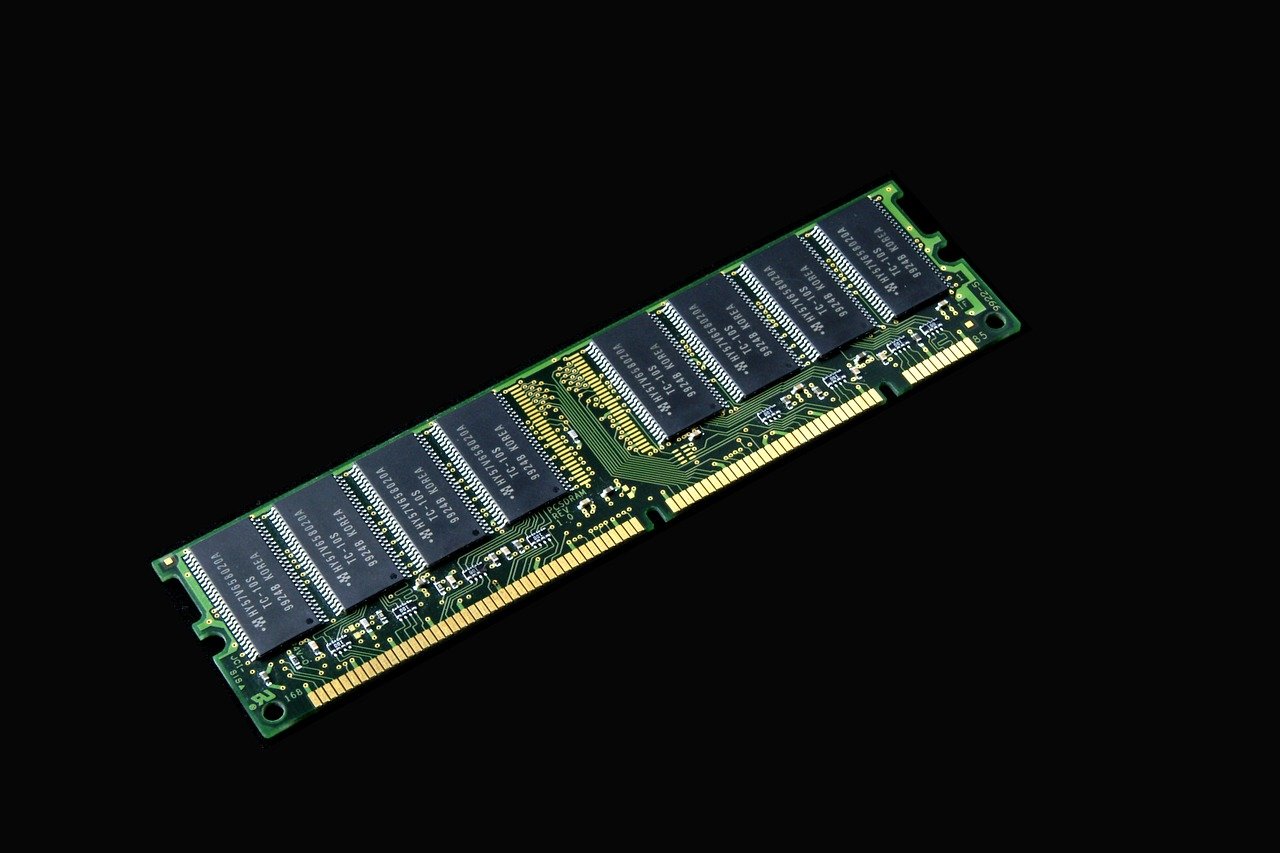
Drug Discovery and Healthcare
The realm of healthcare is on the brink of a revolutionary transformation, thanks to the advent of quantum computing. Imagine a world where drug discovery is not only faster but also more efficient and tailored to individual needs. Quantum computing holds the key to unlocking this potential by simulating complex molecular interactions that are currently beyond the reach of classical computers. This technology could drastically reduce the time it takes to bring new drugs to market, which traditionally spans over a decade and costs billions of dollars. With quantum computing, researchers can model the behavior of molecules at an atomic level, allowing them to predict how different compounds will react within the body.
One of the significant advantages of quantum computing in drug discovery is its ability to analyze vast datasets. For instance, when researchers are searching for new pharmaceuticals, they often need to sift through millions of chemical compounds. Quantum computers can perform these calculations in a fraction of the time it would take classical computers, leading to quicker identification of promising candidates. This speed not only accelerates the process but also enhances the accuracy of predictions regarding a drug's effectiveness and safety.
Additionally, quantum computing can facilitate the development of personalized medicine. By analyzing a patient's genetic makeup and health history, quantum algorithms can help create customized treatment plans that are more effective than one-size-fits-all approaches. This level of precision could significantly improve patient outcomes and reduce the trial-and-error nature of current treatment methodologies.
However, the integration of quantum computing into healthcare is not without its challenges. The technology is still in its infancy, and there are several hurdles to overcome, including the need for robust quantum algorithms and the infrastructure to support them. Researchers are actively working on these issues, but it will take time before quantum computing becomes a staple in drug discovery and healthcare.
In summary, the potential of quantum computing in the field of drug discovery and healthcare is immense. By accelerating the development of new medications and enabling personalized treatment strategies, quantum technology could lead to breakthroughs that enhance the quality of life for countless individuals. As we continue to explore this exciting frontier, we must also prepare for the challenges that lie ahead.
- What is quantum computing? Quantum computing leverages the principles of quantum mechanics to process information in ways that classical computers cannot, offering unprecedented computational power.
- How can quantum computing benefit drug discovery? It can simulate molecular interactions, analyze large datasets rapidly, and facilitate personalized medicine, ultimately speeding up the drug development process.
- Are there any challenges to implementing quantum computing in healthcare? Yes, significant challenges include technical hurdles, high costs, and the need for specialized expertise in quantum technologies.
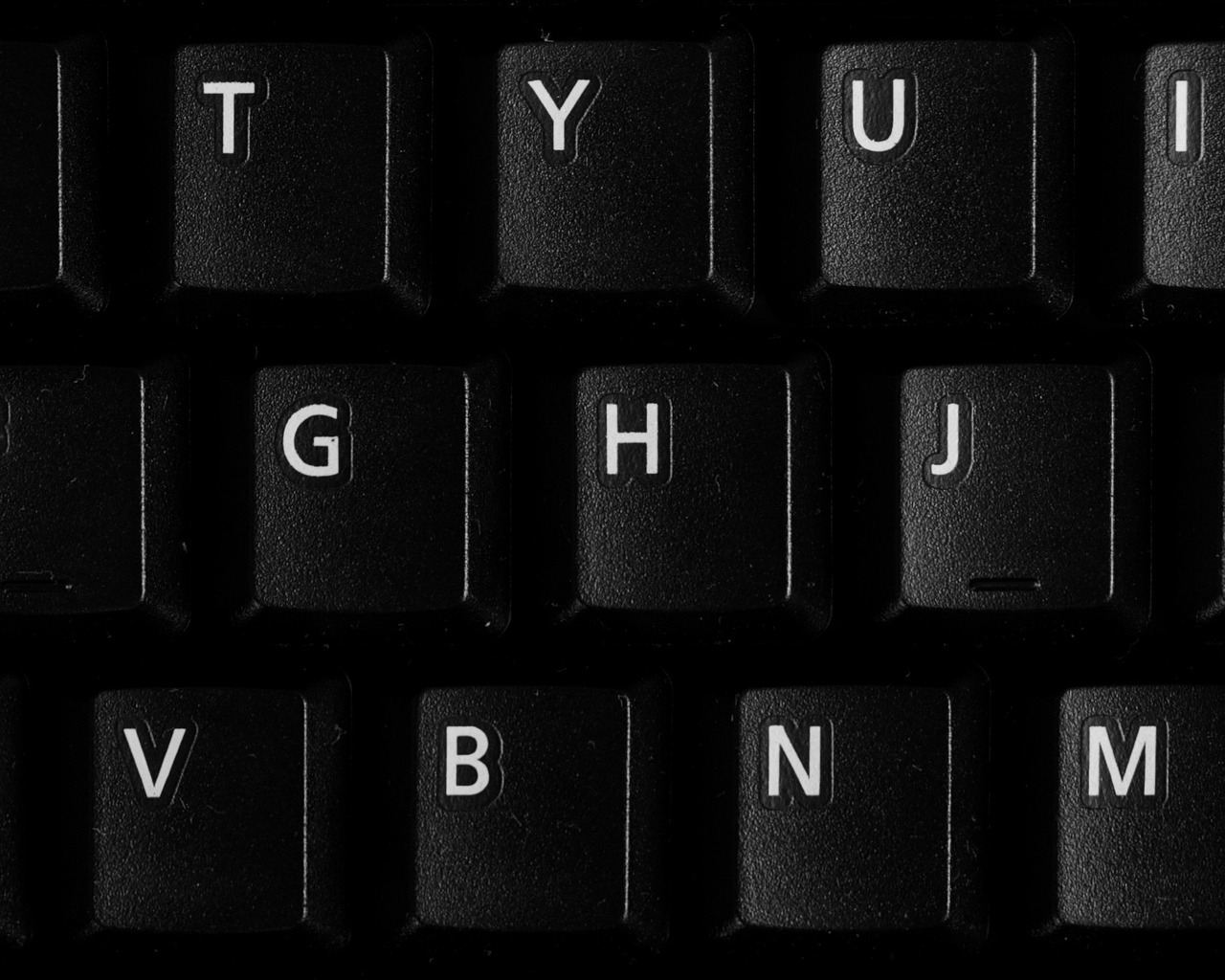
Challenges and Limitations
Despite the **transformative potential** of quantum computing, there are significant challenges and limitations that need to be addressed before it can be fully realized. Building stable and reliable quantum computers is not just a walk in the park; it requires overcoming a multitude of technical hurdles. For instance, one of the primary challenges lies in maintaining qubit coherence. Qubits, the fundamental units of quantum information, are notoriously fragile. They can easily lose their quantum state due to environmental interference, which is often referred to as decoherence. This issue makes it difficult to perform long computations without errors.
Moreover, error correction is another critical aspect that researchers are grappling with. Traditional error correction methods used in classical computing simply do not apply to quantum systems. This is because measuring a qubit can change its state, leading to a paradox in error correction. To tackle this, scientists are developing quantum error correction codes, which, while promising, add layers of complexity and still require extensive resources.
In addition to these technical challenges, there are also substantial cost barriers. The high costs associated with developing and maintaining quantum computing infrastructure can be prohibitive. This includes the need for specialized equipment, such as cryogenic systems to keep superconducting qubits at extremely low temperatures, and highly controlled environments to ensure qubit stability. These expenses limit accessibility, posing a significant barrier for smaller organizations and researchers eager to explore quantum technologies. The following table outlines some of the key challenges and their implications:
Challenge | Description | Implication |
---|---|---|
Qubit Coherence | Qubits lose their quantum state due to environmental interference. | Limits the duration of computations. |
Error Correction | Traditional methods are ineffective; new codes are complex. | Increases computational overhead. |
High Costs | Expensive infrastructure and specialized equipment are required. | Restricts access for smaller entities. |
Furthermore, the need for specialized expertise cannot be overlooked. Quantum computing is a field that requires a deep understanding of quantum mechanics, computer science, and engineering. This combination of skills is not commonly found, creating a talent gap that could slow down progress in the industry. As the demand for quantum computing grows, educational institutions will need to step up and provide programs that equip the next generation of scientists and engineers with the necessary skills.
In conclusion, while the promise of quantum computing is indeed exciting, the road ahead is fraught with challenges. Addressing these technical hurdles, financial constraints, and the need for specialized talent will be crucial for unlocking the full potential of quantum technologies. The journey may be long, but the rewards could be revolutionary, transforming industries and changing the way we solve complex problems.
- What is quantum computing? Quantum computing harnesses the principles of quantum mechanics to process information in ways that classical computers cannot.
- What are qubits? Qubits are the basic units of quantum information, analogous to classical bits but capable of existing in multiple states simultaneously.
- Why is coherence important? Coherence is crucial for maintaining the quantum state of qubits during computations, influencing the accuracy and reliability of quantum operations.
- What industries can benefit from quantum computing? Industries such as finance, healthcare, and cryptography are expected to see significant advancements through the use of quantum computing.
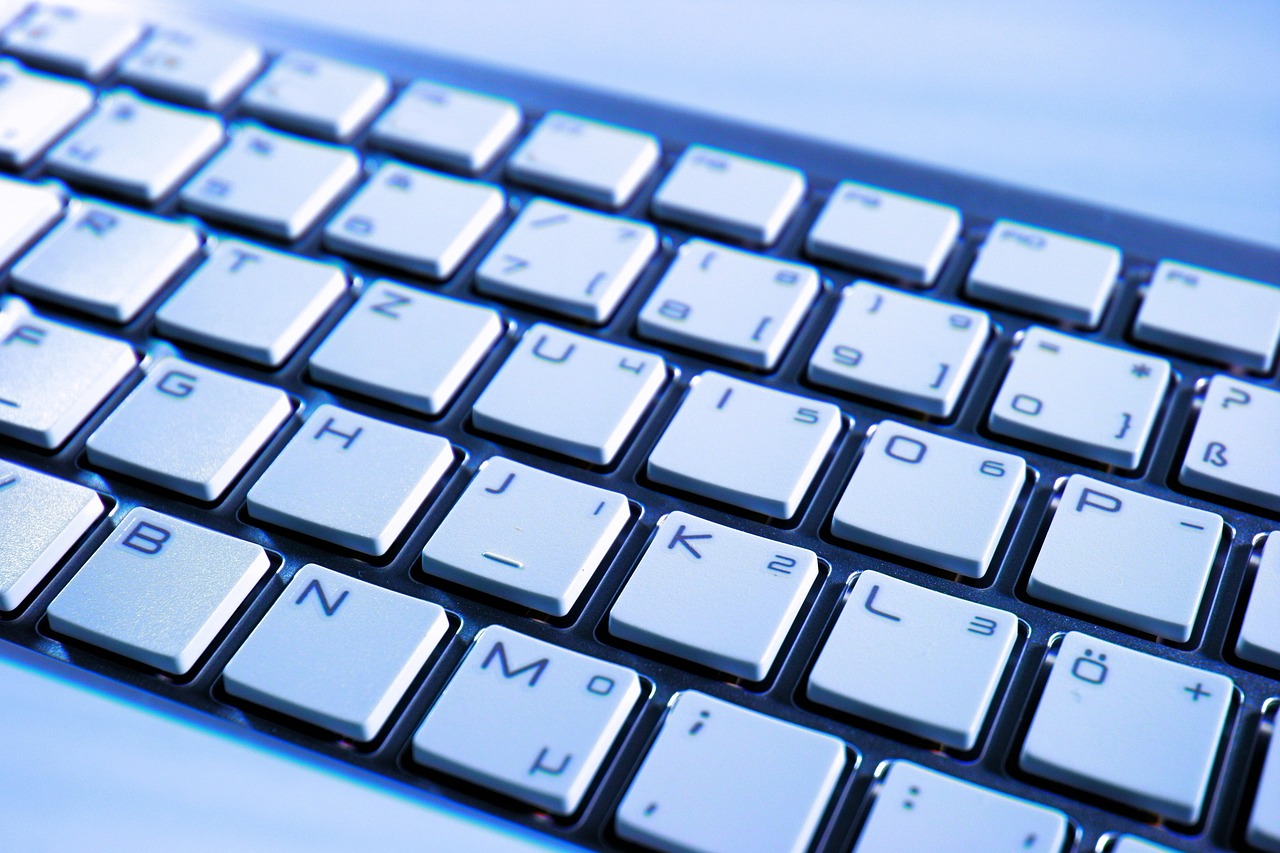
Technical Hurdles
The journey towards realizing the full potential of quantum computing is not without its challenges. As exciting as this technology is, it faces a myriad of that researchers and engineers must overcome to bring quantum computers from the lab to practical use. One of the most pressing issues is qubit coherence. Qubits, the fundamental units of quantum information, must maintain their quantum state long enough to perform calculations. Unfortunately, they are notoriously sensitive to their environment, leading to rapid decoherence that can disrupt computations.
Another significant challenge is error correction. In classical computing, error correction is relatively straightforward; however, in quantum computing, the situation is far more complex. Quantum states cannot be copied due to the no-cloning theorem, making traditional error correction methods ineffective. Researchers are actively exploring various quantum error correction codes, but these solutions often require additional qubits, complicating the architecture and increasing the resource demands.
Furthermore, system integration poses a substantial hurdle. Building a quantum computer involves not just the qubits themselves but also the supporting infrastructure, such as control systems and measurement devices. Integrating these components into a cohesive system that operates reliably is a daunting task. For instance, superconducting qubits require precise microwave pulses for control, while trapped ions need laser systems to manipulate individual ions. The synchronization of these technologies is crucial yet challenging.
To illustrate these technical hurdles more clearly, consider the following table that summarizes some of the key challenges:
Technical Challenge | Description |
---|---|
Qubit Coherence | Maintaining quantum states long enough to perform computations. |
Error Correction | Developing methods to correct errors without disrupting quantum states. |
System Integration | Combining various components into a functional quantum computer. |
Lastly, the need for specialized expertise cannot be overlooked. Quantum computing is a highly interdisciplinary field, requiring knowledge of physics, computer science, and engineering. The current shortage of qualified professionals in this niche area poses another barrier to progress. As the demand for quantum technology grows, so does the need for educational programs and training to equip the next generation of researchers and engineers with the necessary skills.
In summary, while the future of quantum computing is bright, the path forward is littered with technical hurdles that require innovative solutions. Overcoming these challenges will be crucial for unlocking the transformative power of quantum computing across various sectors.
- What is quantum computing? Quantum computing leverages the principles of quantum mechanics to process information in ways that classical computers cannot.
- What are qubits? Qubits are the basic units of quantum information, analogous to bits in classical computing.
- Why is error correction important in quantum computing? Error correction is crucial because quantum states are fragile and susceptible to interference, which can lead to incorrect computations.
- What are some applications of quantum computing? Quantum computing has potential applications in cryptography, drug discovery, optimization problems, and more.
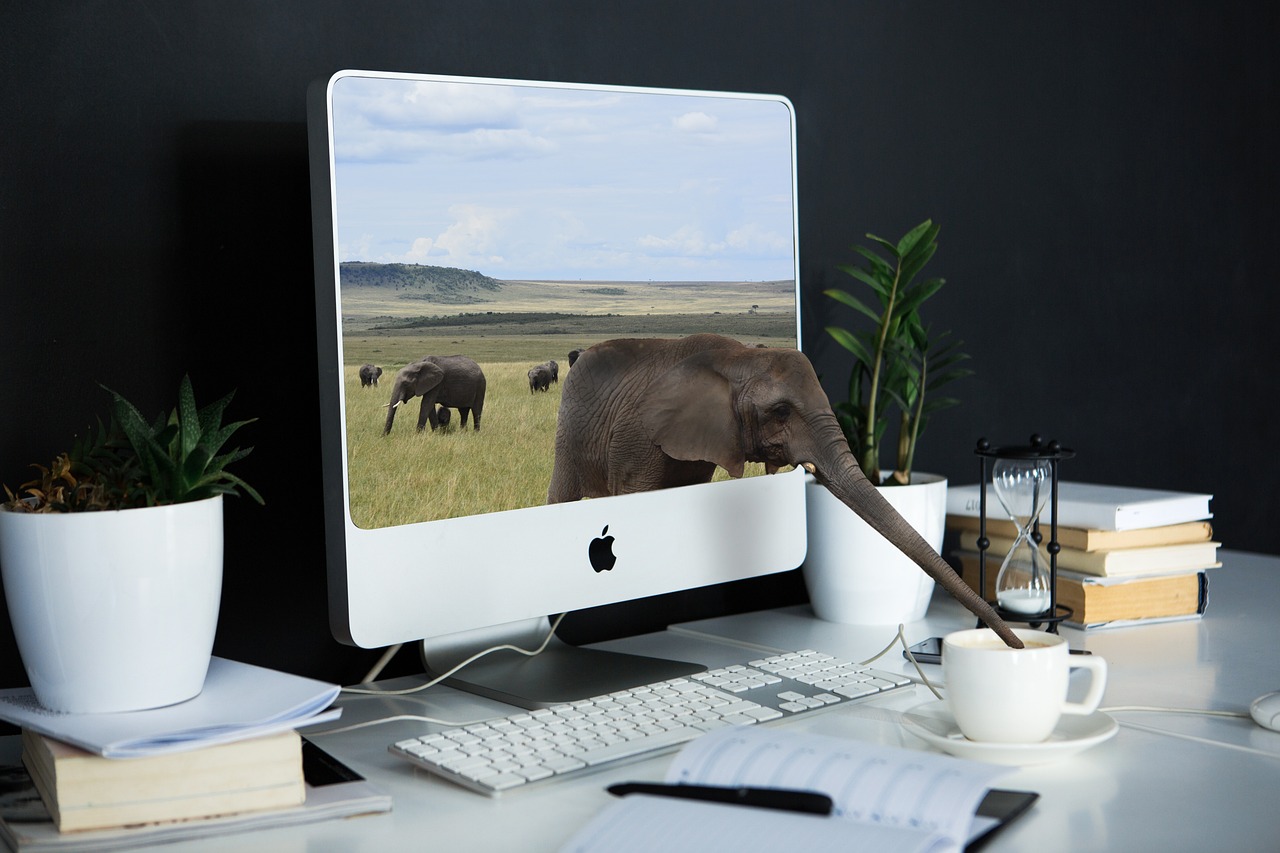
Cost and Accessibility
The journey into the realm of quantum computing is not just about groundbreaking technology; it also comes with a hefty price tag. Imagine trying to buy the latest smartphone, but instead of a few hundred dollars, you’re looking at millions! This is the reality for organizations venturing into quantum computing. The initial investment for quantum hardware, specialized software, and the infrastructure required to support these systems can be astronomical. For instance, the cost of a single quantum computer can range from $10 million to $15 million, making it a luxury that only a handful of tech giants and well-funded research institutions can afford.
Moreover, the operational costs don’t stop at just purchasing the equipment. Maintaining these sophisticated machines requires a team of highly skilled professionals, including quantum physicists, engineers, and software developers. This need for specialized expertise further drives up costs, creating a barrier for smaller companies and startups who may want to explore quantum technologies but find themselves priced out of the market. It’s like wanting to join an exclusive club but realizing the membership fee is beyond your reach!
Accessibility is another significant issue. While large organizations might have the resources to invest in quantum computing, smaller entities often struggle. The disparity in access can lead to a digital divide in technological advancement, where only a select few can leverage the power of quantum computing. In a world where innovation is key, this divide can stifle creativity and progress, leaving smaller players at a disadvantage.
To illustrate the cost implications, let’s take a look at a simple table that outlines the various costs associated with quantum computing:
Cost Category | Estimated Cost |
---|---|
Quantum Hardware | $10 million - $15 million |
Operational Costs (Annual) | $1 million - $3 million |
Research and Development | $500,000 - $2 million |
Specialized Personnel | $200,000 - $500,000 per expert |
As you can see, the financial commitment required for quantum computing is substantial. It’s not just about having the technology; it’s about sustaining it. This reality poses a challenge for many aspiring innovators who might have brilliant ideas but lack the resources to bring them to fruition. In this landscape, collaboration between established companies and startups could be a game-changer, potentially paving the way for shared resources and knowledge that could democratize access to quantum technologies.
In conclusion, while quantum computing holds immense potential to revolutionize various industries, the issues of cost and accessibility must be addressed. Without a concerted effort to make these technologies more affordable and accessible, we risk creating a future where only a select few can harness the true power of quantum computing. The challenge lies in finding innovative solutions that can bridge this gap and ensure that the benefits of quantum advancements are available to all.
- What is quantum computing? Quantum computing is a type of computing that utilizes the principles of quantum mechanics to process information in ways that classical computers cannot.
- Why is quantum computing so expensive? The high costs are due to the sophisticated hardware, specialized personnel required, and the infrastructure needed to support quantum systems.
- How can smaller organizations access quantum computing? Collaborations and partnerships with larger tech firms can provide smaller organizations with access to quantum technologies and resources.
- What industries will benefit the most from quantum computing? Industries such as finance, healthcare, and cryptography are expected to see significant advancements through quantum computing.
Frequently Asked Questions
- What is quantum computing?
Quantum computing is a revolutionary technology that utilizes the principles of quantum mechanics to process information in ways that classical computers cannot. This means it can solve complex problems at unprecedented speeds, making it a game-changer for various industries.
- How do superconducting qubits work?
Superconducting qubits operate by using superconducting materials to create qubits that can be manipulated rapidly. They are known for their speed and ability to integrate into larger quantum systems, but they face challenges related to coherence and error rates that researchers are actively trying to overcome.
- What are the main applications of quantum computing?
Quantum computing has the potential to revolutionize fields such as cryptography, drug discovery, and optimization problems. For instance, it can break traditional encryption methods, leading to the development of quantum-resistant algorithms, and it can accelerate drug discovery by simulating molecular interactions.
- What challenges does quantum computing face?
Despite its promise, quantum computing faces significant hurdles, including technical challenges like qubit coherence and error correction, as well as high costs that limit accessibility. These factors contribute to the slow pace of its widespread adoption.
- Can quantum computers break current encryption methods?
Yes, quantum computers have the potential to break many traditional encryption methods, which is why there is a strong push towards developing quantum-resistant algorithms to secure sensitive information in our increasingly digital world.
- How is quantum computing impacting healthcare?
In healthcare, quantum computing can dramatically speed up drug discovery processes by accurately simulating molecular interactions. This could lead to more effective treatments and personalized medicine, all while reducing the time it takes to bring new drugs to market.
- What is the future of quantum computing?
The future of quantum computing is bright but uncertain. While ongoing research is addressing technical and financial challenges, the technology is expected to become more practical and accessible over the coming years, potentially transforming various sectors.