The Science of Genetic Engineering and Its Applications
Welcome to the fascinating world of genetic engineering, a field that holds the keys to unlocking the mysteries of life itself. Imagine being able to alter the very blueprint of an organism, tweaking its DNA to enhance desirable traits or eliminate harmful ones. Sounds like science fiction, right? Well, it’s not! This article explores the fundamental principles of genetic engineering, its various techniques, and the diverse applications across medicine, agriculture, and environmental science. We will also highlight its potential and the ethical considerations that come with such powerful technology.
At its core, genetic engineering involves manipulating an organism's DNA to alter its characteristics. This process can be as simple as adding a single gene or as complex as replacing entire sequences of DNA. The history of genetic engineering is filled with milestones that have shaped our understanding and capabilities in this field. From the discovery of the double helix structure of DNA by Watson and Crick to the first successful cloning of a mammal, Dolly the sheep, each breakthrough has paved the way for more advanced techniques and applications.
There are several techniques utilized in genetic engineering, each with its unique advantages and applications. Some of the most notable methods include CRISPR, gene cloning, and transgenic technology. These tools have revolutionized the way scientists approach genetic modification, making it faster, cheaper, and more precise than ever before. Understanding these techniques is crucial for grasping the potential impact of genetic engineering on our world.
One of the most groundbreaking advancements in genetic engineering is the CRISPR-Cas9 technology. This method allows for precise edits to an organism's DNA, enabling scientists to add, remove, or alter genetic material with incredible accuracy. Imagine having a pair of molecular scissors that can cut DNA at specific locations, allowing for targeted modifications. This revolutionary technique has opened up a world of possibilities in genetic research and therapies. For instance, it holds promise for correcting genetic disorders, enhancing crop resilience, and even fighting infectious diseases.
The applications of CRISPR in medicine are vast and varied. Researchers are exploring its potential in treating genetic disorders such as cystic fibrosis and sickle cell anemia. Clinical trials are underway to assess its effectiveness in combating cancers and viral infections. For example, a recent trial aimed at using CRISPR to treat patients with beta-thalassemia has shown promising results, raising hopes for a future where genetic diseases can be effectively managed or even cured.
However, with great power comes great responsibility. The ability to edit human genes raises significant ethical questions. Should we have the right to alter the genetic makeup of future generations? What are the potential consequences of "designer babies"? These moral dilemmas necessitate a robust regulatory framework to ensure that genetic engineering is used responsibly and ethically. Society must engage in open dialogues about the implications of these technologies to navigate the complexities they present.
Another fascinating aspect of genetic engineering is the creation of transgenic organisms. These are organisms that have been genetically modified to contain genes from other species. This technique is widely used in agriculture to develop crops that can withstand pests, diseases, and environmental stresses. Additionally, transgenic animals are being researched for their potential to produce pharmaceuticals and enhance food production. The implications of this technology are profound, as it can lead to increased food security and sustainable agricultural practices.
Genetic engineering has significantly transformed agriculture, enabling the development of crops with improved traits such as pest resistance and drought tolerance. These advancements are crucial in addressing global challenges like food security and climate change. For instance, genetically modified (GM) crops have been engineered to require fewer pesticides, benefiting both farmers and the environment. The adoption of these crops has led to increased yields and reduced agricultural losses, showcasing the potential of genetic engineering to revolutionize food production.
Genetically modified crops have gained widespread acceptance and adoption worldwide due to their numerous benefits. These crops are designed to be more resilient, nutritious, and efficient. However, their cultivation is not without controversy. Concerns about long-term health effects, environmental impact, and corporate control over seeds have sparked heated debates. Regulatory challenges also arise as different countries navigate the complexities of GM crop approval and labeling.
The introduction of genetically engineered species can have profound effects on ecosystems. While there are potential benefits, such as reduced pesticide use and increased crop yields, there are also risks to biodiversity. The displacement of native species and the potential for unintended consequences in ecosystems are significant concerns. It is essential to conduct thorough assessments and long-term studies to understand the ecological implications of introducing genetically modified organisms into the environment.
Looking ahead, the future of genetic engineering holds immense potential for innovation across various fields. As technology continues to evolve, we can expect advancements that could lead to breakthroughs in medicine, agriculture, and environmental conservation. Imagine a world where genetic diseases are eradicated, crops can thrive in harsh climates, and ecosystems are restored through targeted genetic interventions. The possibilities are endless, but they also come with responsibilities that society must carefully consider.
- What is genetic engineering? Genetic engineering is the manipulation of an organism's DNA to alter its characteristics.
- How does CRISPR work? CRISPR works like molecular scissors, allowing precise edits to DNA at specific locations.
- What are the applications of genetic engineering in medicine? Genetic engineering can be used to treat genetic disorders, cancers, and infectious diseases.
- Are genetically modified crops safe? While many studies support their safety, ongoing research and regulation are crucial for monitoring their impact.
- What ethical concerns are associated with genetic engineering? Ethical concerns include the potential for designer babies, long-term effects, and environmental impacts.
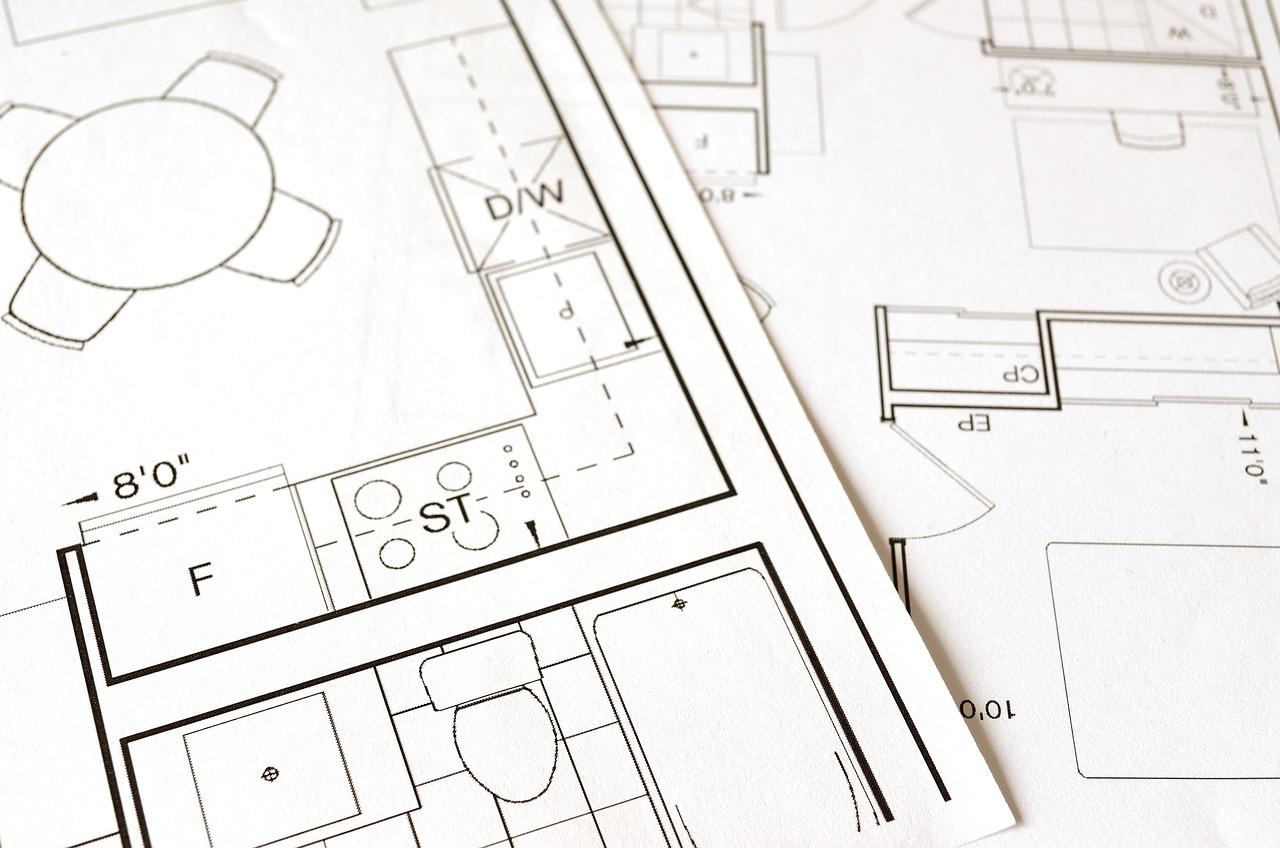
Understanding Genetic Engineering
Genetic engineering is a fascinating field that delves into the very blueprint of life—DNA. Imagine being able to edit the genetic code of an organism, much like editing a document on your computer. This manipulation allows scientists to alter the characteristics of living organisms, creating possibilities that were once confined to the realm of science fiction. But how did we get here? The journey of genetic engineering is paved with groundbreaking discoveries and technological advancements that have shaped our understanding of biology.
At its core, genetic engineering involves the modification of an organism's genome, which is the complete set of genes or genetic material present in a cell or organism. This process can be used to enhance desirable traits, such as increasing crop yields or developing new medical therapies. The techniques employed in genetic engineering are diverse, ranging from traditional methods like selective breeding to cutting-edge technologies like CRISPR-Cas9.
Historically, the roots of genetic engineering can be traced back to the early 20th century with the discovery of DNA's structure by Watson and Crick. This monumental breakthrough laid the groundwork for understanding how genes function and how they can be manipulated. Fast forward to the 1970s, when scientists first successfully cloned a gene, marking a pivotal moment in the field. Since then, the landscape of genetic engineering has evolved rapidly, leading to significant milestones such as the first genetically modified organism (a bacterium) in 1973 and the creation of transgenic plants in the 1980s.
As we explore the principles of genetic engineering, it's essential to recognize the various techniques that have emerged. Here are some key methods:
- Gene Cloning: This technique involves creating copies of a specific gene, allowing researchers to study its function and use it for various applications.
- CRISPR-Cas9: A revolutionary tool that enables precise editing of DNA sequences, making it easier and more efficient to modify genes.
- Transgenic Technology: This method involves inserting genes from one organism into another, creating organisms with new traits.
Each of these techniques has its unique applications and implications, paving the way for innovations in medicine, agriculture, and environmental science. However, with great power comes great responsibility. As we harness the potential of genetic engineering, we must also navigate the ethical considerations that arise from our ability to manipulate life at such a fundamental level. The discussion surrounding genetic engineering is not just about the science; it also encompasses the moral dilemmas and societal impacts that come with it.
In summary, understanding genetic engineering is about appreciating the intricate dance between science, technology, and ethics. As we continue to unlock the secrets of DNA, the possibilities seem endless, offering a glimpse into a future where we can potentially eradicate diseases, enhance food security, and even combat climate change. The journey has just begun, and the next chapters in the story of genetic engineering promise to be just as exciting.
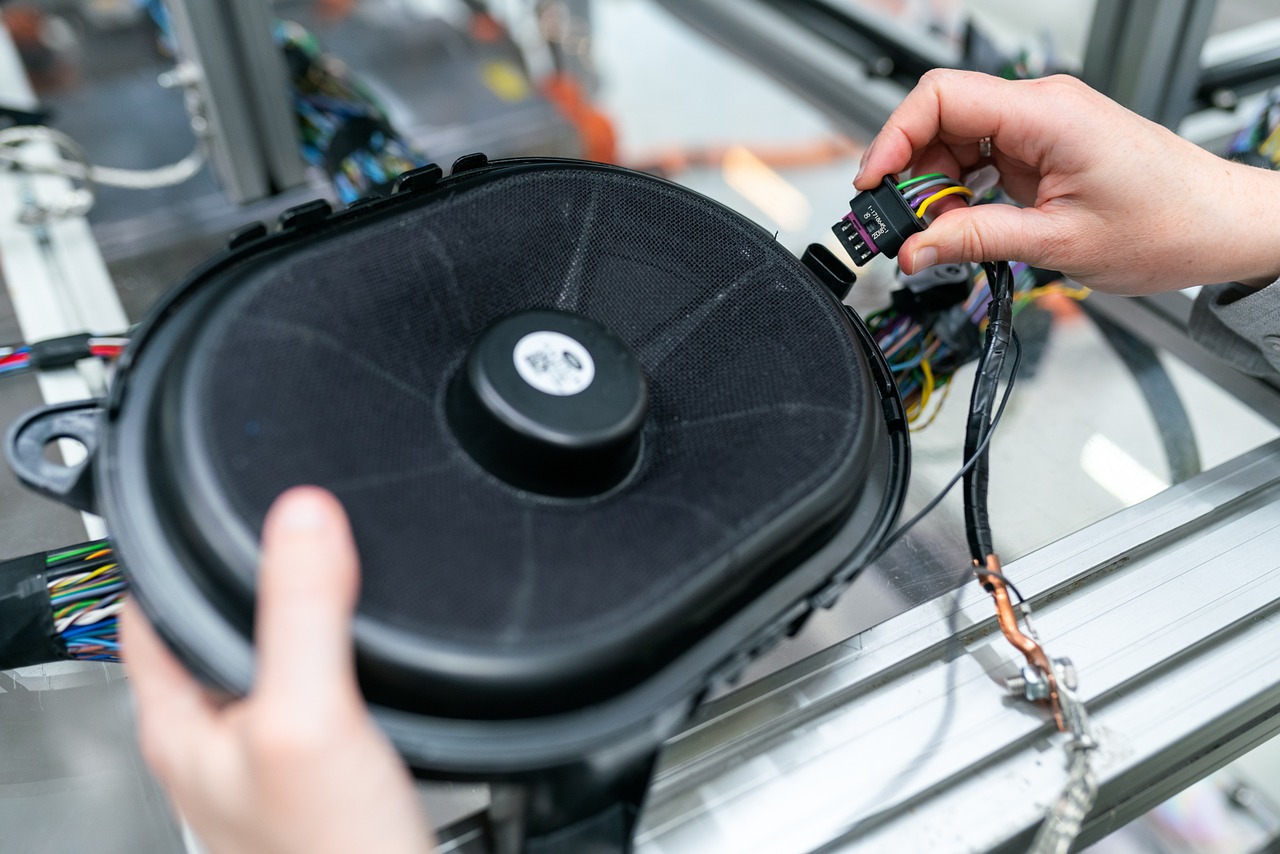
Techniques in Genetic Engineering
Genetic engineering is a fascinating field that employs various techniques to manipulate the genetic material of organisms. The toolbox available to geneticists today is diverse and powerful, allowing for precise modifications that can lead to groundbreaking advancements in medicine, agriculture, and environmental science. Understanding these techniques is essential for grasping the potential and implications of genetic engineering.
One of the most revolutionary techniques in genetic engineering is CRISPR-Cas9. This technology enables scientists to edit genes with remarkable precision, akin to using a word processor to correct a typo in a document. With CRISPR, researchers can target specific sequences of DNA and make alterations, whether it’s inserting a new gene, deleting a faulty one, or modifying existing genes. The simplicity and efficiency of CRISPR have made it a go-to method for genetic modifications in various organisms, from bacteria to plants and even animals.
Another significant technique is gene cloning, which involves creating copies of specific genes. This process allows scientists to study the function of genes in detail and produce proteins for therapeutic use. For instance, gene cloning has been instrumental in producing insulin for diabetes treatment. By inserting the human insulin gene into bacteria, researchers can generate large quantities of insulin, making it accessible and affordable for patients in need.
Additionally, the concept of transgenic technology has made waves in both agriculture and medicine. This technique involves inserting genes from one species into another, creating organisms that exhibit traits from both. For example, scientists have developed transgenic crops that are resistant to pests or can thrive in harsh environmental conditions. These innovations not only enhance crop yields but also contribute to sustainable farming practices.
To better understand these techniques, here’s a brief comparison:
Technique | Description | Applications |
---|---|---|
CRISPR-Cas9 | Precise gene editing tool that allows targeted modifications. | Genetic research, treatment of genetic disorders, agriculture. |
Gene Cloning | Creating copies of specific genes for study and production. | Insulin production, gene therapy, research. |
Transgenic Technology | Inserting genes from one species into another. | Development of genetically modified crops, research. |
The significance of these techniques cannot be overstated. They have opened new avenues for research and have the potential to solve some of the world's most pressing challenges, such as food security and disease management. However, as we harness these powerful tools, it's crucial to consider the ethical implications and ensure that we navigate this complex landscape responsibly.
- What is genetic engineering? Genetic engineering is the direct manipulation of an organism's DNA to alter its characteristics.
- What are the main techniques used in genetic engineering? The main techniques include CRISPR-Cas9, gene cloning, and transgenic technology.
- How does CRISPR-Cas9 work? CRISPR-Cas9 works by using a guide RNA to locate a specific DNA sequence, allowing the Cas9 enzyme to cut the DNA at that location for editing.
- What are transgenic organisms? Transgenic organisms are those that have had genes from another species inserted into their genome.
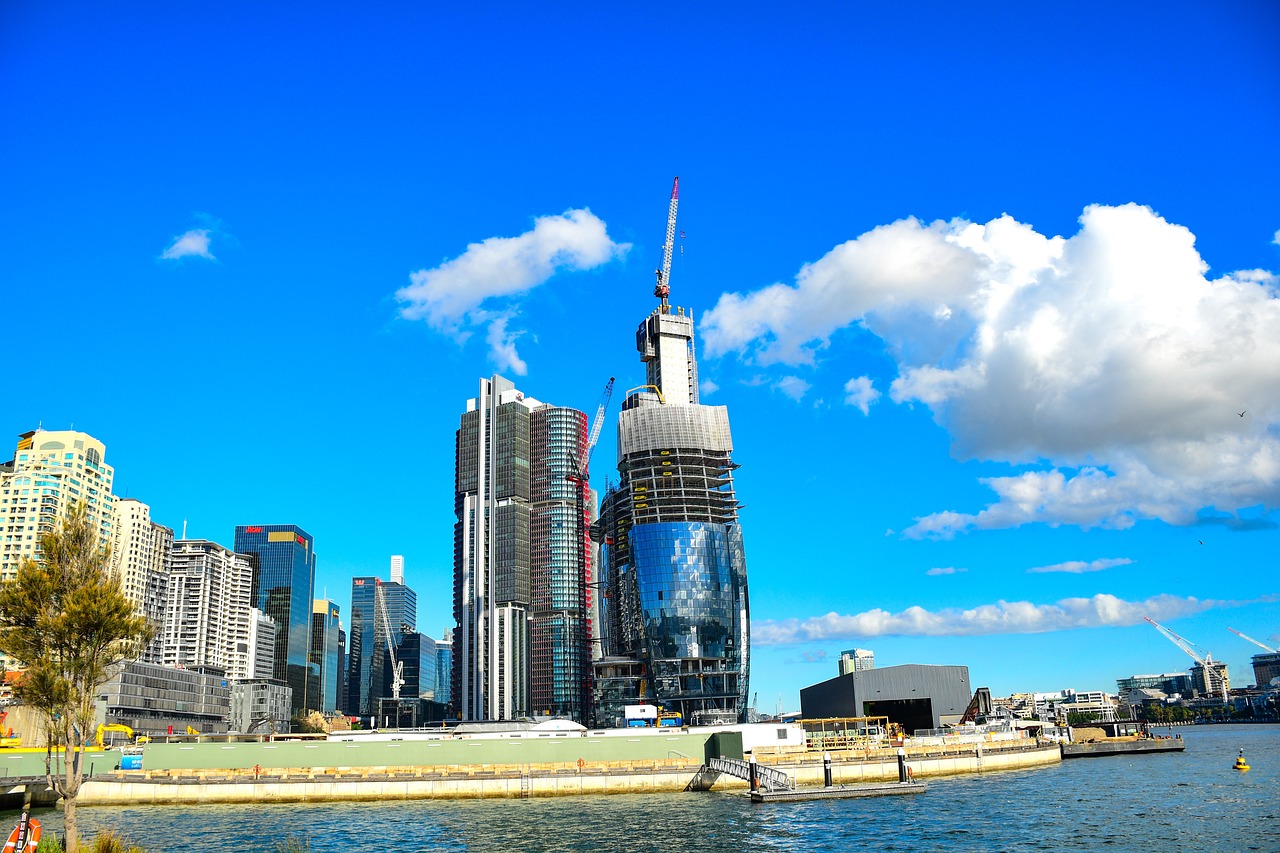
CRISPR-Cas9 Technology
CRISPR-Cas9 technology has taken the world of genetic engineering by storm, and for good reason! Imagine having the ability to edit the genetic code of living organisms with the precision of a word processor. That's what CRISPR-Cas9 allows scientists to do. This groundbreaking tool, derived from a natural defense mechanism in bacteria, enables researchers to target specific sequences in DNA and make precise modifications. The mechanism is relatively straightforward: it uses a guide RNA to locate the desired DNA sequence, and then the Cas9 enzyme acts like molecular scissors to cut the DNA at that exact spot. This opens up a whole new world of possibilities in genetics!
One of the most exciting aspects of CRISPR-Cas9 is its versatility. It’s not just a tool for basic research; it has far-reaching implications in various fields. Here are a few key applications:
- Gene Therapy: Correcting genetic defects by directly editing the faulty genes.
- Cancer Research: Understanding the genetic basis of tumors and potentially developing targeted therapies.
- Infectious Diseases: Developing treatments that can target and eliminate viral DNA from infected cells.
As we delve deeper into the potential of CRISPR-Cas9, it’s essential to highlight some remarkable case studies that showcase its transformative power. For instance, researchers have been able to create genetically modified mice that can resist certain types of cancer. This not only aids in understanding the disease but also paves the way for innovative treatments. Additionally, scientists have been working on using CRISPR to tackle genetic disorders like sickle cell anemia, with promising results emerging from clinical trials.
However, with great power comes great responsibility. The implications of CRISPR-Cas9 technology extend beyond just scientific advancement; they raise important ethical questions. As we gain the ability to edit human DNA, we must ponder the moral dilemmas surrounding such actions. Should we be allowed to edit the genes of embryos? What are the long-term effects of these modifications on future generations? These questions are not just academic; they have real-world implications that society must grapple with.
In summary, CRISPR-Cas9 technology is a revolutionary tool that has the potential to change the landscape of genetic engineering. Its precise editing capabilities open doors to numerous applications in medicine, agriculture, and beyond. Yet, as we stand on the brink of this genetic revolution, it is crucial to navigate the ethical considerations that accompany such profound advancements.
1. What is CRISPR-Cas9?
CRISPR-Cas9 is a genetic engineering tool that allows for precise editing of DNA by cutting it at specific locations, enabling the addition or removal of genetic material.
2. What are the main applications of CRISPR-Cas9?
Its main applications include gene therapy, cancer research, and the development of treatments for infectious diseases.
3. Are there ethical concerns surrounding CRISPR-Cas9?
Yes, there are significant ethical concerns, particularly regarding gene editing in humans, potential long-term effects, and the implications for future generations.
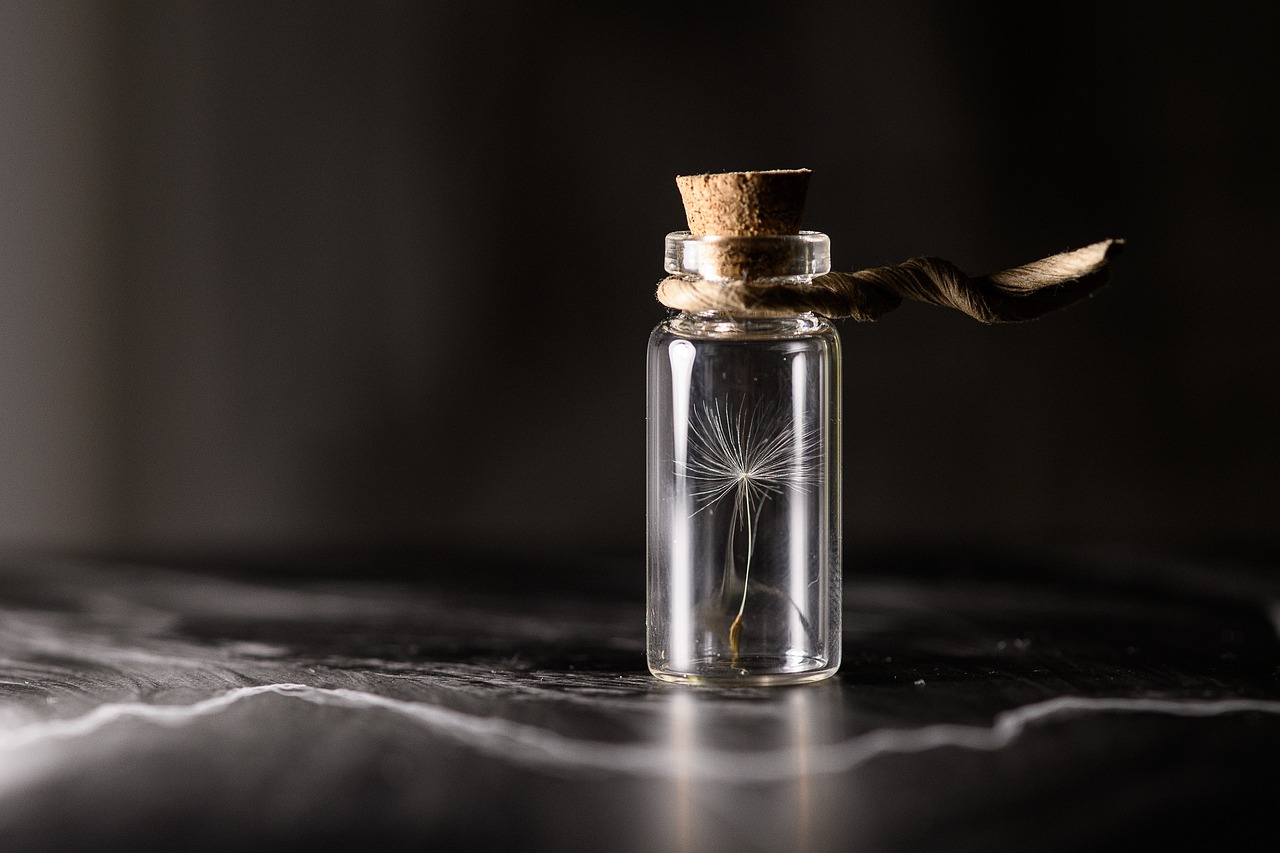
Applications in Medicine
When we talk about the applications of genetic engineering in medicine, it's like opening a treasure chest filled with possibilities that could change the way we treat diseases. Imagine being able to edit out genetic disorders or develop targeted therapies for cancer using the very building blocks of life! This is not just science fiction; it's happening right now, thanks to groundbreaking technologies like CRISPR.
One of the most promising areas is the treatment of genetic disorders. For instance, conditions such as sickle cell anemia and cystic fibrosis are caused by specific mutations in our DNA. Researchers are now exploring how to use CRISPR to correct these mutations. In a recent clinical trial, scientists successfully utilized CRISPR to modify the genes of patients with sickle cell disease, leading to significant improvements in their health. This is a game-changer, demonstrating that we can potentially eliminate genetic diseases at their source.
Moreover, the implications of genetic engineering extend to combating infectious diseases. The rapid development of vaccines, particularly during the COVID-19 pandemic, showcased how genetic engineering can expedite the creation of effective treatments. For instance, mRNA vaccines, which instruct cells to produce a harmless piece of the virus, have proven to be incredibly effective. This technology could pave the way for future vaccines against other diseases, such as HIV or malaria, by enabling a quicker response to emerging pathogens.
But it doesn't stop there. Gene therapy is another exciting application, where healthy genes are introduced into a patient's cells to replace or repair faulty ones. This method has shown promise in treating conditions like hemophilia and certain types of blindness. Imagine being able to restore sight to someone who has been visually impaired for years simply by correcting a genetic defect!
However, it's crucial to discuss the ethical implications that come with these advancements. The ability to edit genes raises questions about designer babies, genetic privacy, and the potential for unintended consequences. As we explore these cutting-edge applications, we must also tread carefully and consider the long-term effects on individuals and society as a whole.
In summary, the applications of genetic engineering in medicine are vast and varied, holding the promise of revolutionary treatments for some of the most challenging health issues we face today. As we continue to unlock the secrets of our DNA, the potential for innovation in medical science is boundless.
- What is genetic engineering? Genetic engineering is the direct manipulation of an organism's DNA to alter its characteristics.
- How does CRISPR work in medicine? CRISPR allows scientists to make precise edits to DNA, which can help correct genetic disorders or develop targeted therapies.
- What are some diseases that can be treated with genetic engineering? Diseases like sickle cell anemia, cystic fibrosis, and certain cancers are being targeted with genetic engineering techniques.
- Are there ethical concerns with genetic engineering? Yes, ethical concerns include the potential for designer babies, genetic privacy, and long-term effects on society.
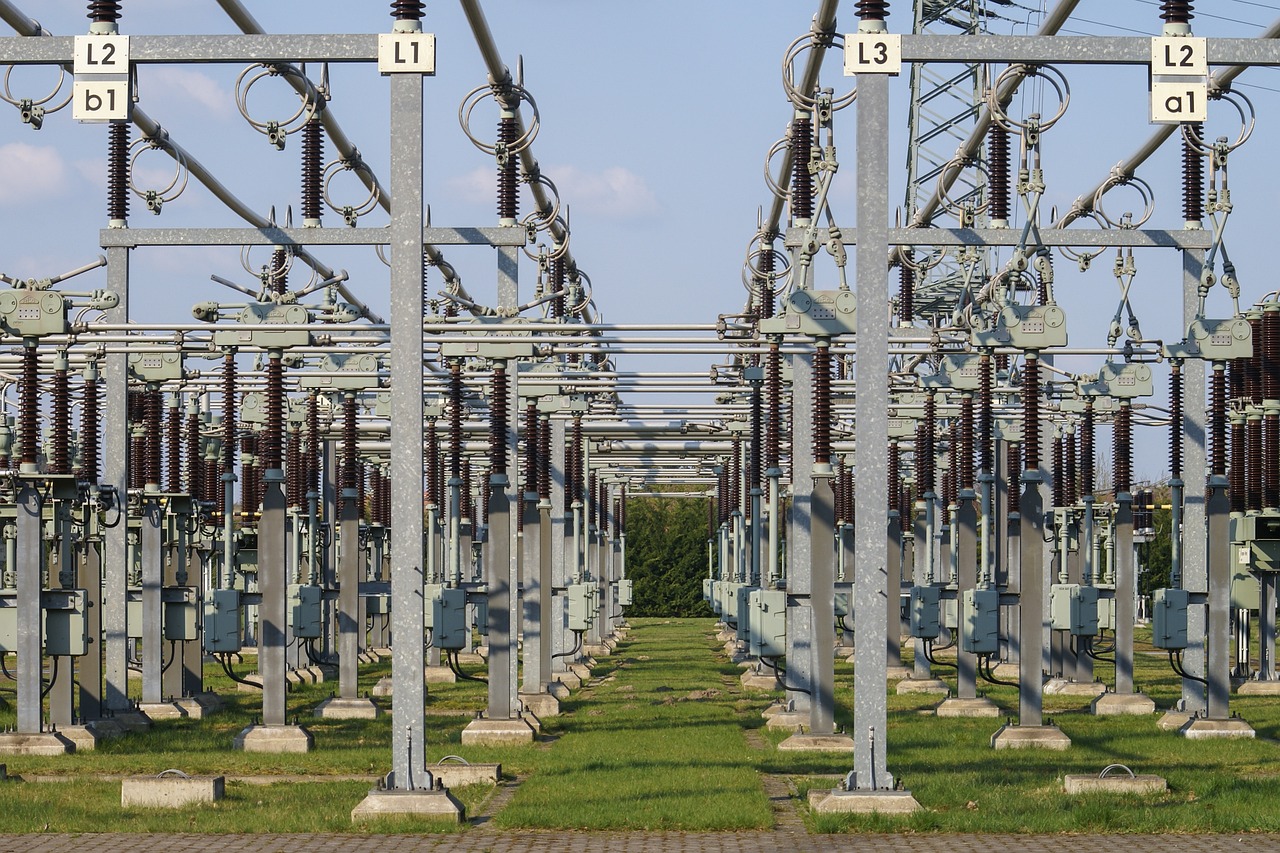
Ethical Considerations
The advent of genetic engineering, especially with the introduction of powerful tools like CRISPR, has sparked a heated debate surrounding the ethical implications of manipulating the very fabric of life. As we stand on the brink of a new era in biotechnology, we must ask ourselves: what are the moral boundaries we should respect? The ability to edit genes presents a Pandora's box of possibilities and challenges that society must navigate carefully.
One of the primary ethical dilemmas revolves around the concept of “playing God.” Are we overstepping our bounds by altering genetic material that has evolved over millions of years? This question becomes particularly poignant when discussing the potential for human germline editing, where changes could be passed down to future generations. The implications of such actions are profound, raising concerns about unintended consequences, such as the emergence of new genetic diseases or unforeseen health issues.
Moreover, the accessibility of genetic engineering technologies poses another ethical challenge. Currently, there is a significant disparity in access to these advancements. Wealthier nations and individuals may benefit disproportionately, leading to a new form of genetic inequality. This disparity raises questions about justice and equity in healthcare and agricultural practices. Should we allow genetic enhancements to be available only to those who can afford them? The potential for a “genetic divide” could exacerbate existing social inequalities.
Additionally, there are concerns regarding the environmental impact of genetically engineered organisms. While the intention behind creating transgenic crops is often to improve yields and reduce pesticide use, the long-term effects on ecosystems remain uncertain. For instance, the introduction of genetically modified organisms (GMOs) into the wild could disrupt local biodiversity, leading to the extinction of native species or the emergence of superweeds resistant to herbicides. This raises the question: how do we balance innovation with the preservation of our planet’s ecosystems?
To navigate these complex ethical waters, regulatory frameworks must evolve alongside technological advancements. Governments and organizations need to establish clear guidelines that prioritize safety, transparency, and public engagement. Engaging with the public through discussions and consultations can help demystify genetic engineering and foster a more informed debate about its implications.
In summary, while genetic engineering holds incredible promise for advancing medicine, agriculture, and environmental science, it also brings forth a myriad of ethical considerations that cannot be overlooked. As we forge ahead into this uncharted territory, we must proceed with caution, ensuring that our actions reflect our values and respect for life in all its forms.
- What is genetic engineering? Genetic engineering is the direct manipulation of an organism's DNA to alter its characteristics.
- What are the ethical concerns surrounding genetic engineering? Ethical concerns include the potential for "playing God," genetic inequality, and environmental impacts.
- How does CRISPR technology work? CRISPR allows for precise edits to DNA, making it a powerful tool in genetic engineering.
- What are transgenic organisms? Transgenic organisms are created by inserting genes from one species into another, often used in agriculture and research.
- What regulations exist for genetic engineering? Regulations vary by country, but they often focus on safety, efficacy, and ethical considerations.

Transgenic Organisms
Transgenic organisms are a fascinating product of genetic engineering, created by inserting genes from one species into the genome of another. This innovative technique allows scientists to imbue plants, animals, and even microorganisms with desirable traits that are not naturally found in their species. Imagine a world where crops can withstand drought, or where livestock can grow faster and be more resistant to diseases. This is not science fiction; it’s the reality of transgenic technology!
The process of creating transgenic organisms typically involves identifying a gene of interest, isolating it, and then introducing it into the target organism's DNA. This can be done using various methods, such as Agrobacterium-mediated transformation for plants or microinjection for animals. Once the gene is integrated into the host genome, it can be expressed, resulting in the manifestation of the new trait.
One of the most notable applications of transgenic organisms is in agriculture. For instance, crops like Bt corn and Roundup Ready soybeans have been engineered to express traits that enhance their resilience against pests and herbicides, respectively. These advancements have led to increased yields and reduced reliance on chemical pesticides, which can be beneficial for both farmers and the environment.
In addition to agriculture, transgenic organisms have significant applications in medicine and research. For example, scientists have developed transgenic mice that carry human genes, which are invaluable for studying genetic diseases and testing new therapies. These mice serve as models that mimic human conditions, allowing researchers to gain insights into disease mechanisms and potential treatments.
However, the creation and use of transgenic organisms are not without controversy. Many people raise concerns about the potential risks associated with genetically modified organisms (GMOs), such as unforeseen ecological impacts and ethical considerations regarding animal welfare. For instance, the introduction of transgenic crops into the environment could lead to gene flow—the transfer of engineered genes to wild relatives, potentially disrupting local ecosystems.
To address these concerns, regulatory frameworks have been established in various countries to ensure that transgenic organisms are thoroughly evaluated for safety and efficacy before they are released into the market. These regulations aim to balance the potential benefits of genetic engineering with the need to protect human health and the environment.
In summary, transgenic organisms represent a remarkable intersection of science and technology, offering solutions to some of the most pressing challenges in agriculture and medicine. As research continues to advance, the potential for transgenic organisms to contribute positively to society remains vast, but it is imperative to approach this powerful tool with caution and ethical consideration.
- What are transgenic organisms? Transgenic organisms are those that have had genes from another species inserted into their DNA, resulting in new traits.
- How are transgenic organisms created? They are created through methods like Agrobacterium-mediated transformation or microinjection, where specific genes are introduced into the target organism's genome.
- What are some applications of transgenic organisms? They are widely used in agriculture to produce pest-resistant crops, in medicine for developing disease models, and in research for studying genetic functions.
- Are there risks associated with transgenic organisms? Yes, potential risks include ecological impacts, gene flow to wild species, and ethical concerns regarding animal welfare.
- How are transgenic organisms regulated? Regulatory frameworks are in place in many countries to evaluate the safety and efficacy of transgenic organisms before they are approved for use.

Applications in Agriculture
Genetic engineering has dramatically transformed the landscape of agriculture, ushering in a new era of farming that promises to address some of the world’s most pressing food security challenges. Imagine a world where crops can thrive in harsh climates, resist pests without the need for harmful pesticides, and yield more food than ever before. This is not just a dream; it is a reality made possible through the innovative techniques of genetic engineering.
One of the most significant advancements in agricultural biotechnology is the development of Genetically Modified Organisms (GMOs). These are organisms whose genetic material has been altered using genetic engineering techniques to express desired traits. For instance, scientists have successfully created crops that are resistant to specific pests, reducing the need for chemical pesticides. This not only benefits farmers by lowering costs but also contributes to a healthier environment by minimizing chemical runoff into ecosystems.
Another remarkable application is the development of crops that are tolerant to extreme environmental conditions, such as drought or salinity. With climate change wreaking havoc on traditional farming practices, these genetically engineered crops can survive and produce food in areas previously considered inhospitable for agriculture. This resilience is crucial for ensuring food availability in regions facing water scarcity or adverse weather conditions.
To illustrate the impact of these advancements, consider the following table that highlights some of the key genetically modified crops and their benefits:
Crop | Trait | Benefit |
---|---|---|
Corn | Pest Resistance | Reduced pesticide use and increased yield |
Soybeans | Herbicide Tolerance | Lower weed competition and easier management |
Rice | Nutritional Enhancement (Golden Rice) | Increased Vitamin A content to combat malnutrition |
Potatoes | Bruise Resistance | Longer shelf life and reduced waste |
However, while the benefits of genetically engineered crops are substantial, they are not without controversy. Many people express concerns regarding the long-term effects of GMOs on health and the environment. Critics argue that the introduction of genetically modified species could disrupt local ecosystems, leading to unintended consequences. It is crucial to approach these innovations with a balanced perspective, weighing both the potential benefits and the risks involved.
Moreover, the regulatory landscape surrounding GMOs varies significantly across countries, complicating their adoption and commercialization. Some regions embrace the technology wholeheartedly, while others impose strict regulations or outright bans, often fueled by public apprehension. This disparity can create challenges for farmers looking to utilize the latest advancements in genetic engineering.
As we look to the future, the role of genetic engineering in agriculture will likely continue to expand. With ongoing research and development, we can expect to see even more innovative solutions that address food security, sustainability, and environmental conservation. The potential is immense, and as we harness the power of genetic engineering, we must also remain vigilant about the ethical implications and ecological impacts of our choices.
- What are genetically modified crops?
Genetically modified crops are plants whose DNA has been altered using biotechnology to introduce desirable traits such as pest resistance or drought tolerance. - Are GMOs safe for human consumption?
Numerous studies have shown that GMOs are safe to eat, but ongoing research and regulatory oversight are essential to ensure their safety. - How do GMOs impact the environment?
GMOs can reduce the need for chemical pesticides and fertilizers, but they may also pose risks to biodiversity and ecosystems if not managed properly. - What is the future of genetic engineering in agriculture?
The future looks promising, with advancements expected in crop resilience, nutritional enhancement, and sustainable farming practices.
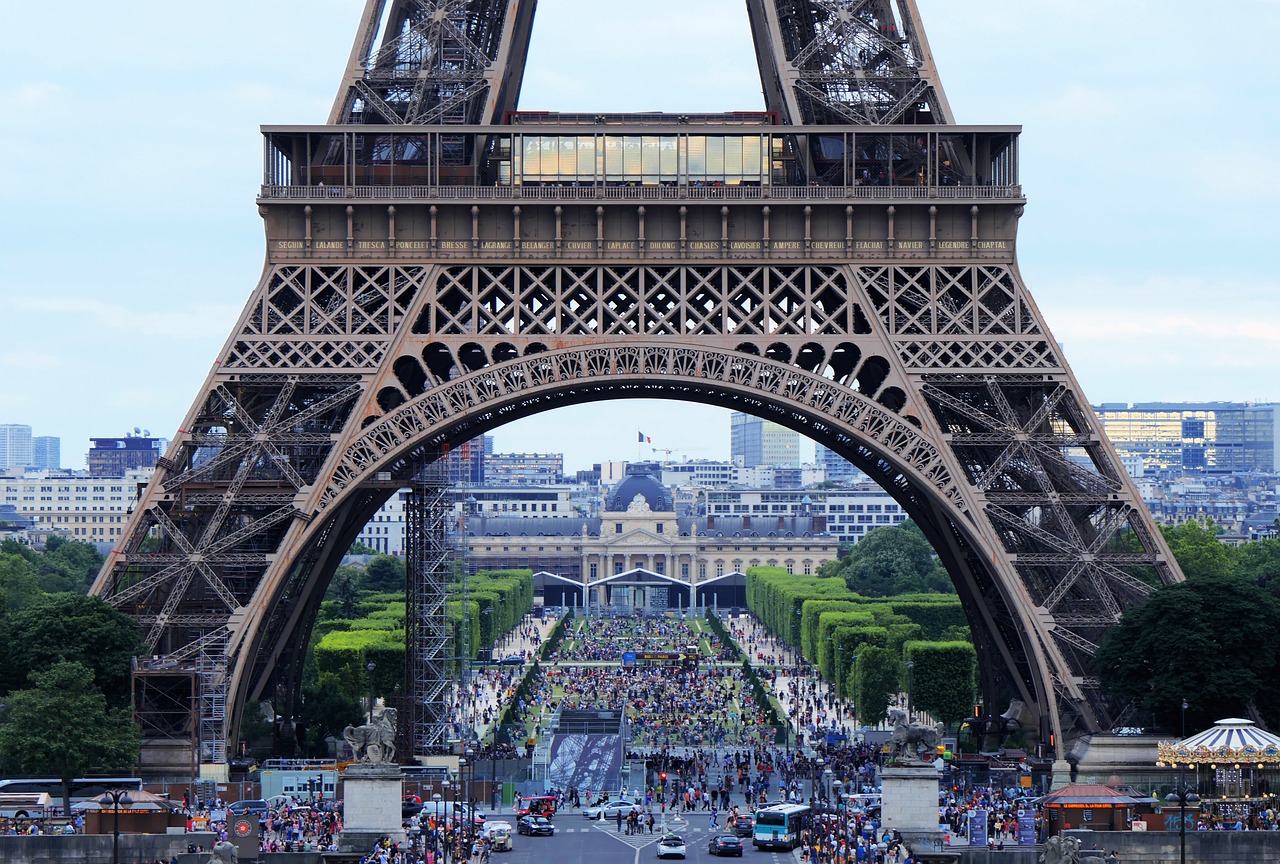
Genetically Modified Crops
Genetically modified crops, often referred to as GMOs (genetically modified organisms), have become a significant part of modern agriculture. These crops are engineered to possess specific traits that enhance their growth, resistance to pests, and overall yield. Imagine a world where farmers can grow crops that withstand droughts and pests without relying heavily on chemical pesticides—this is not just a dream, but a reality made possible by genetic engineering.
The benefits of genetically modified crops are numerous, and they have been adopted worldwide for their potential to improve food security. For instance, crops like Bt cotton and Bt corn are modified to express a protein from the bacterium Bacillus thuringiensis, which is toxic to certain pests but safe for human consumption. This not only reduces the need for chemical insecticides but also leads to higher yields for farmers. In fact, studies have shown that farmers growing GM crops can experience a significant increase in their profits due to reduced losses from pests and lower input costs.
However, the adoption of genetically modified crops is not without controversy. Critics argue that GMOs may pose risks to human health and the environment. For example, there are concerns about the potential for allergenicity and the long-term effects of consuming genetically modified foods. Additionally, the possibility of cross-breeding between GM crops and wild relatives raises questions about biodiversity and ecosystem stability. To address these concerns, regulatory frameworks have been established in many countries to evaluate the safety and environmental impact of GMOs before they are approved for commercial use.
Here’s a quick overview of some of the key advantages and challenges associated with genetically modified crops:
Advantages | Challenges |
---|---|
Increased yield potential | Potential health risks |
Reduced pesticide use | Environmental concerns |
Improved nutritional content | Ethical considerations |
Drought resistance | Market resistance |
As we look to the future, the role of genetically modified crops in addressing global food challenges becomes increasingly important. With a growing population and the effects of climate change, the ability to produce resilient and high-yielding crops is vital. Innovations in genetic engineering will likely continue to evolve, leading to new varieties that can thrive in extreme conditions while providing essential nutrients to populations around the world.
In conclusion, genetically modified crops represent a powerful tool in the agricultural toolbox. While they offer tremendous benefits, it’s essential to continue monitoring their impact and addressing public concerns to foster a balanced approach to food production. The dialogue surrounding GMOs is crucial, as it shapes the future of agriculture and food security for generations to come.
- What are genetically modified crops? Genetically modified crops are plants whose DNA has been altered using genetic engineering techniques to exhibit desired traits such as pest resistance or drought tolerance.
- Are genetically modified crops safe to eat? Extensive research and regulatory assessments have shown that approved GM crops are safe for human consumption.
- How do genetically modified crops benefit farmers? They can lead to higher yields, reduced pesticide use, and lower production costs, ultimately increasing profitability.
- What are the environmental impacts of GM crops? While GM crops can reduce the need for chemical inputs, concerns about biodiversity and ecosystem balance remain important considerations.

Impact on Biodiversity
The introduction of genetically engineered species into ecosystems is a topic that sparks intense debate among scientists, environmentalists, and the general public alike. On one hand, genetic engineering holds the promise of enhancing agricultural productivity and combating food insecurity. On the other hand, it raises significant concerns about its potential impact on biodiversity. But what does this really mean for our planet? Let’s dive into the complexities of how genetically modified organisms (GMOs) can affect biodiversity.
One of the primary concerns is that genetically modified crops may outcompete native species. When a genetically engineered plant is introduced into an environment, it can sometimes thrive better than its non-modified counterparts. This competitive advantage can lead to a decrease in the population of native plants. Over time, this can result in a shift in the ecosystem, as the diversity of plant life diminishes. This phenomenon is often referred to as "ecological homogenization," where unique local species are replaced by a limited number of genetically engineered varieties.
Moreover, the use of genetically modified crops can have cascading effects on the entire food web. For instance, if a plant is engineered to be pest-resistant, it might alter the dynamics of insect populations that rely on it for food. This can lead to a decline in the insects that feed on these crops, which in turn affects the birds and other animals that depend on those insects for survival. It's a delicate balance, and disrupting one part of the system can lead to unforeseen consequences.
To better understand these impacts, let’s consider a few key points:
- Genetic Drift: There is a risk that genes from genetically modified crops can transfer to wild relatives, creating hybrid plants that may behave unpredictably in nature.
- Loss of Genetic Diversity: As farmers increasingly adopt genetically modified crops, traditional varieties may be neglected, leading to a loss of genetic diversity that is crucial for resilience against pests and diseases.
- Impact on Pollinators: The introduction of GMOs can also affect pollinator populations, which are vital for many crops and wild plants. Changes in plant composition can lead to declines in pollinator species, impacting food production and ecosystem health.
However, it’s not all doom and gloom. There are arguments that genetically engineered crops can actually contribute to biodiversity by reducing the need for chemical pesticides, which can be harmful to various forms of life. Additionally, some genetically modified plants are designed to thrive in harsh conditions, potentially allowing for the cultivation of crops in areas previously deemed unsuitable for agriculture. This could lead to the preservation of natural habitats elsewhere.
In conclusion, the impact of genetic engineering on biodiversity is a double-edged sword. While it offers innovative solutions for food production and environmental challenges, it also poses risks that must be carefully managed. As we continue to explore the potential of genetic engineering, it’s crucial that we weigh its benefits against its ecological consequences to ensure a sustainable future for all species on our planet.
Q1: What is biodiversity?
Biodiversity refers to the variety of life forms on Earth, including the diversity of species, ecosystems, and genetic variations within species. It’s essential for ecosystem resilience and human survival.
Q2: How do genetically modified organisms affect biodiversity?
GMOs can impact biodiversity by outcompeting native species, altering food webs, and potentially leading to a loss of genetic diversity. However, they can also reduce the need for harmful pesticides and allow for agriculture in challenging environments.
Q3: Are there regulations in place for genetically modified organisms?
Yes, many countries have regulations governing the development and release of GMOs to ensure environmental safety and assess potential risks to biodiversity.
Q4: Can genetically modified crops help in conservation efforts?
In some cases, yes. By increasing agricultural efficiency and reducing the need for land conversion, GMOs can help preserve natural habitats and support conservation efforts.

Future Perspectives
The field of genetic engineering is on the brink of a revolutionary leap forward, much like the dawn of the Internet transformed communication. As we stand at this exciting crossroads, the potential for innovation seems boundless. Imagine a world where genetic disorders can be corrected before birth, where crops can withstand extreme climate conditions, and where diseases can be eradicated before they even manifest. The future is not just a distant dream; it's a rapidly approaching reality.
One of the most thrilling aspects of genetic engineering is the ongoing research into new techniques and applications. For instance, advancements in synthetic biology are paving the way for creating entirely new organisms designed to perform specific tasks, such as breaking down pollutants in the environment. This could revolutionize how we approach environmental restoration and pollution management. With the right tools and understanding, we might even engineer microbes that can produce biofuels, reducing our reliance on fossil fuels and mitigating climate change.
Moreover, the integration of artificial intelligence with genetic engineering is another frontier that holds immense promise. AI can analyze vast datasets to identify genetic patterns and predict outcomes of genetic modifications. This synergy between AI and genetic engineering could streamline the development of new therapies and agricultural innovations, making the process faster and more efficient. Imagine a future where a simple algorithm could suggest the best genetic modifications for a specific plant based on climate data, soil conditions, and pest threats. It’s like having a personal genetic advisor at your fingertips!
However, with great power comes great responsibility. As we explore these new frontiers, ethical considerations will become increasingly significant. Questions surrounding the use of genetic engineering in humans, especially in relation to designer babies, will require careful deliberation. Society must grapple with the implications of such capabilities, ensuring that advancements are used for the greater good and not for creating inequalities based on genetic enhancements.
In agriculture, the future of genetically modified organisms (GMOs) is equally promising. As global populations continue to rise, the demand for food will escalate. Genetic engineering can play a crucial role in developing crops that are not only more nutritious but also capable of thriving in less than ideal conditions. For example, researchers are working on crops that can grow in saline soils or require significantly less water. These innovations are essential for food security, especially in regions that are already facing the brunt of climate change.
To summarize the potential future advancements in genetic engineering, consider the following table that outlines key areas of development:
Area of Development | Potential Impact |
---|---|
Gene Editing Techniques | Enhanced precision in modifying genes, leading to fewer off-target effects. |
Synthetic Biology | Creation of new organisms for environmental and industrial applications. |
AI Integration | Streamlined research and development processes in genetic modifications. |
Ethical Frameworks | Guidelines to ensure responsible use of genetic engineering technologies. |
As we look ahead, it's clear that the journey of genetic engineering is just beginning. The innovations on the horizon not only promise to enhance our lives but also challenge us to think critically about the ethical implications of our actions. The future is bright, but it requires a collective effort to navigate it wisely.
- What is genetic engineering? Genetic engineering is the direct manipulation of an organism's DNA to alter its characteristics.
- What are some applications of genetic engineering? Applications include medicine (gene therapy), agriculture (genetically modified crops), and environmental science (bioremediation).
- What is CRISPR-Cas9? CRISPR-Cas9 is a revolutionary gene-editing technology that allows for precise modifications to DNA.
- Are there ethical concerns with genetic engineering? Yes, ethical concerns include the potential for designer babies, biodiversity impacts, and the implications of modifying human genes.
Frequently Asked Questions
- What is genetic engineering?
Genetic engineering is the process of altering the DNA of an organism to change its characteristics. This can involve adding, removing, or modifying genes to achieve desired traits, making it a powerful tool in various fields such as medicine, agriculture, and environmental science.
- How does CRISPR-Cas9 work?
CRISPR-Cas9 is a revolutionary gene-editing technology that allows scientists to make precise changes to DNA. It works by using a guide RNA to locate a specific sequence in the genome, and then the Cas9 enzyme cuts the DNA at that location, enabling the addition or removal of genetic material.
- What are some applications of genetic engineering in medicine?
In medicine, genetic engineering has the potential to treat genetic disorders, cancers, and infectious diseases. For example, researchers are exploring the use of CRISPR to correct mutations that cause diseases like sickle cell anemia and to develop targeted therapies for various cancers.
- Are there ethical concerns surrounding genetic engineering?
Yes, the power of genetic engineering, particularly with technologies like CRISPR, raises significant ethical questions. Issues include the potential for "designer babies," the long-term effects of gene editing, and the need for regulatory frameworks to govern its use in humans.
- What are transgenic organisms?
Transgenic organisms are created by inserting genes from one species into another. This technique is widely used in agriculture to develop crops that are resistant to pests or harsh environmental conditions, and it also has applications in medicine and research.
- How do genetically modified crops impact agriculture?
Genetically modified (GM) crops have transformed agriculture by enhancing traits like pest resistance and drought tolerance. While they can increase yields and reduce the need for chemical pesticides, they also spark debates about safety, environmental impact, and biodiversity.
- What are the potential risks of genetic engineering on biodiversity?
The introduction of genetically engineered species can disrupt ecosystems and affect biodiversity. While there are potential benefits, such as increased food production, there are concerns about the long-term ecological impacts and the risk of GM organisms outcompeting native species.
- What does the future hold for genetic engineering?
The future of genetic engineering is promising, with advancements expected to revolutionize fields like medicine, agriculture, and environmental conservation. Innovations may lead to new therapies for diseases, improved crop varieties, and solutions to pressing environmental challenges.