How New Discoveries in Physics are Shaping Our Understanding of the Universe
The universe is a vast and mysterious place, filled with wonders that challenge our understanding of reality. With each passing year, scientists are making groundbreaking discoveries that reshape our comprehension of the cosmos. From the tiniest particles to the largest galaxies, the field of physics is at the forefront of exploring these enigmas. This article delves into the latest breakthroughs in physics, examining how they influence our understanding of the universe, the implications of these findings, and what they mean for future scientific exploration. Buckle up, because the ride through the cosmos is about to get thrilling!
Quantum mechanics has revolutionized our understanding of the microscopic world. Imagine a realm where particles can exist in multiple states at once, where they can be entangled across vast distances, and where the act of observation can change the outcome of an experiment. This is the strange yet fascinating world of quantum mechanics. It provides profound insights into particle behavior and the fundamental nature of reality. The implications of these discoveries are not just theoretical; they have paved the way for groundbreaking technologies such as quantum computing and quantum cryptography. These technologies promise to enhance our computational power and secure our communications in ways we could only dream of a few decades ago.
As we gaze into the cosmos, recent discoveries in astrophysics have transformed our perspective on cosmic phenomena. The identification of black holes and the detection of gravitational waves have opened new avenues for understanding the universe's structure and evolution. Each discovery is like peeling back layers of an onion, revealing deeper truths about the fabric of space and time. Through these advancements, we are not just observers of the universe; we are beginning to decode its secrets.
Black holes are among the most intriguing and enigmatic objects in the universe. They challenge our understanding of gravity and spacetime, leading to groundbreaking research that could reveal the fundamental laws governing the universe. These cosmic giants exert such a strong gravitational pull that not even light can escape their grasp. This begs the question: what lies beyond the event horizon? The mysteries of black holes could hold the key to understanding singularities and the nature of the universe itself.
The Event Horizon Telescope (EHT) has provided unprecedented images of black holes, enhancing our grasp of their properties and behaviors. In 2019, the EHT collaboration unveiled the first-ever image of a black hole's event horizon, located in the galaxy M87. This monumental achievement confirmed several theoretical predictions in Einstein's general relativity and has set the stage for further exploration of these cosmic phenomena.
The detection of gravitational waves has opened a new window into the universe, allowing scientists to observe cosmic events like colliding black holes and neutron stars. These ripples in spacetime, first detected in 2015, have reshaped our understanding of astrophysical phenomena. They provide a novel way to study the universe, akin to listening to the music of the cosmos, where each wave tells a story of celestial collisions and cosmic dances.
Despite making up about 95% of the universe's total mass-energy content, dark matter and dark energy remain elusive and poorly understood. Dark matter acts like a cosmic glue, holding galaxies together, while dark energy drives the accelerated expansion of the universe. The quest to uncover their properties is akin to searching for the missing pieces of a cosmic puzzle, and physicists are working tirelessly to understand their roles in cosmic expansion and structure formation.
The Standard Model describes the fundamental particles and forces in the universe, forming the bedrock of particle physics. However, recent findings challenge its completeness, prompting physicists to search for new theories beyond its framework. It's like having a map that only shows part of the terrain; there is so much more to discover that could redefine our understanding of the universe.
The discovery of the Higgs boson was a monumental milestone in particle physics. This particle is responsible for giving mass to other particles, confirming the mechanism that underpins the structure of matter. However, its discovery has also raised questions about the universe's fundamental structure and whether there are more particles waiting to be discovered.
Exploring theories beyond the Standard Model, such as supersymmetry and string theory, aims to address unanswered questions in physics. These theories propose a more unified understanding of the universe, suggesting that there may be hidden dimensions and particles yet to be observed. The pursuit of these theories is like venturing into uncharted territory, where each step could lead to revelations that redefine our understanding of existence.
The future of theoretical physics holds immense promise for new discoveries that could redefine our understanding of the universe. Ongoing research into quantum gravity, multiverse theories, and the nature of time itself is pushing the boundaries of what we know. As scientists continue to explore these complex ideas, we stand on the brink of a new era of discovery, where the mysteries of the universe may finally be unveiled.
- What is quantum mechanics? Quantum mechanics is a fundamental theory in physics that describes the behavior of matter and energy at the smallest scales, such as atoms and subatomic particles.
- What are black holes? Black holes are regions in space where the gravitational pull is so strong that nothing, not even light, can escape from them.
- What is dark matter? Dark matter is a form of matter that does not emit light or energy, making it invisible and detectable only through its gravitational effects on visible matter.
- What is the Standard Model? The Standard Model is a theoretical framework in particle physics that describes the fundamental particles and forces that govern the universe.
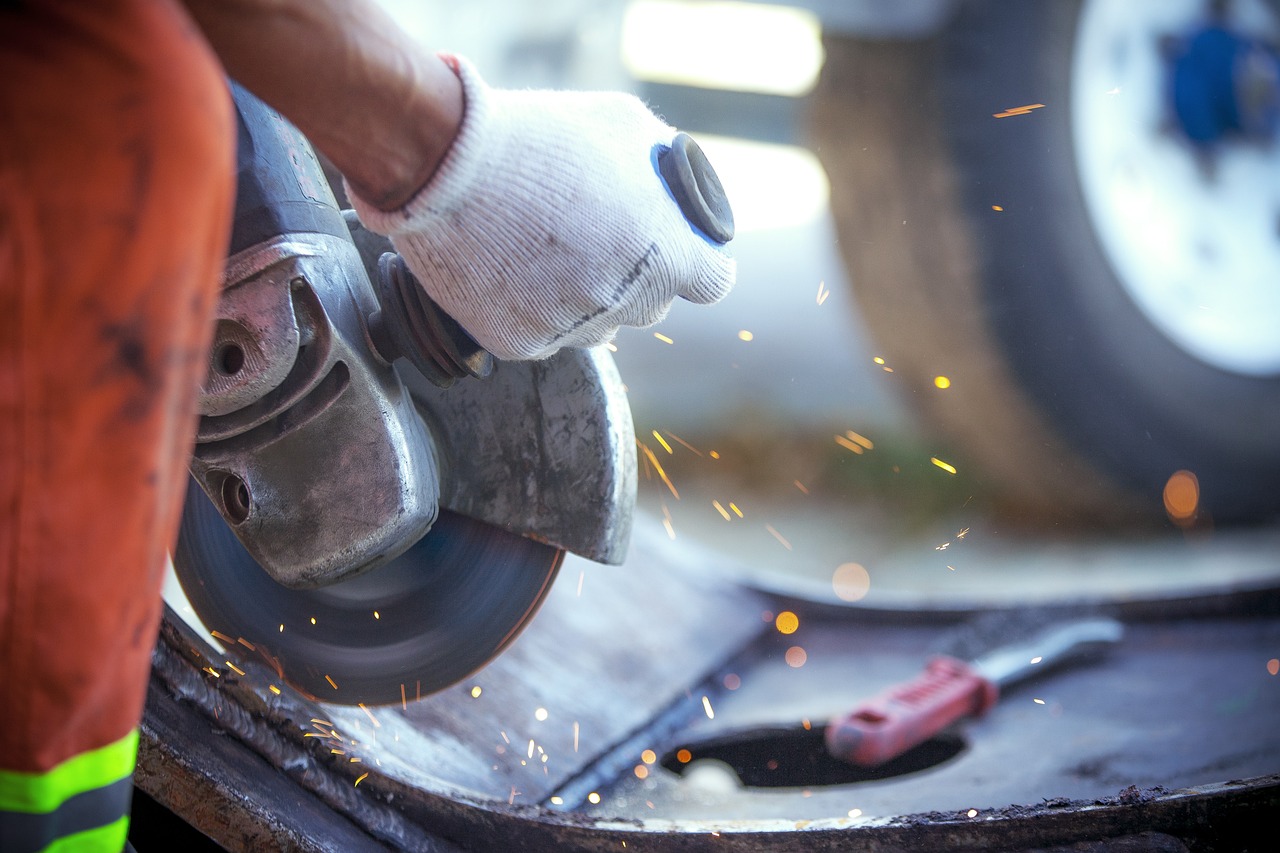
The Role of Quantum Mechanics
Quantum mechanics has fundamentally reshaped our understanding of the microscopic world, revealing a realm that operates under rules vastly different from our everyday experiences. Imagine a universe where particles can exist in multiple states at once, where they can be entangled over vast distances, and where observation itself can alter the outcome of an event. It’s like stepping into a surreal dream where the normal laws of physics seem to bend and twist in unexpected ways.
At the heart of quantum mechanics lies the concept of superposition, which suggests that particles can exist in multiple states simultaneously until they are measured. This principle is not merely theoretical; it has profound implications for technology. For instance, quantum computing harnesses this principle to perform computations at speeds unimaginable with classical computers. Instead of using bits that are either 0 or 1, quantum computers utilize qubits that can be both at the same time, exponentially increasing processing power.
Another fascinating aspect is quantum entanglement, where particles become intertwined in such a way that the state of one instantly influences the state of another, regardless of the distance separating them. This phenomenon has sparked discussions about the very nature of reality and has potential applications in quantum cryptography, promising secure communication channels that are theoretically immune to eavesdropping.
To illustrate the impact of quantum mechanics, consider the following table that highlights some of its revolutionary applications:
Application | Description |
---|---|
Quantum Computing | Uses qubits for faster processing, enabling complex calculations and problem-solving. |
Quantum Cryptography | Provides secure communication by leveraging the principles of quantum mechanics. |
Quantum Teleportation | Transfers quantum states between particles over distance without physical movement. |
Quantum Sensors | Offers unprecedented precision in measurements, useful in various scientific fields. |
As we delve deeper into the quantum realm, we realize that the implications of these discoveries extend beyond technology. They challenge our perceptions of reality itself. Questions arise: What does it mean to observe something? How does consciousness interact with the physical world? These inquiries not only fuel scientific exploration but also inspire philosophical debates that have persisted for centuries.
In summary, quantum mechanics is not just a branch of physics; it is a doorway into a universe that is far more intricate and interconnected than we previously imagined. As we continue to unravel its mysteries, we stand on the brink of a new era in science, one that promises to enhance our understanding of the universe and our place within it.
- What is quantum mechanics? Quantum mechanics is a fundamental theory in physics that describes the behavior of matter and energy at the smallest scales, such as atoms and subatomic particles.
- How does quantum mechanics affect technology? Quantum mechanics has led to the development of technologies such as quantum computing, quantum cryptography, and advanced sensors, transforming various industries.
- What is quantum entanglement? Quantum entanglement is a phenomenon where two or more particles become linked, such that the state of one particle instantly influences the state of the other, regardless of the distance between them.
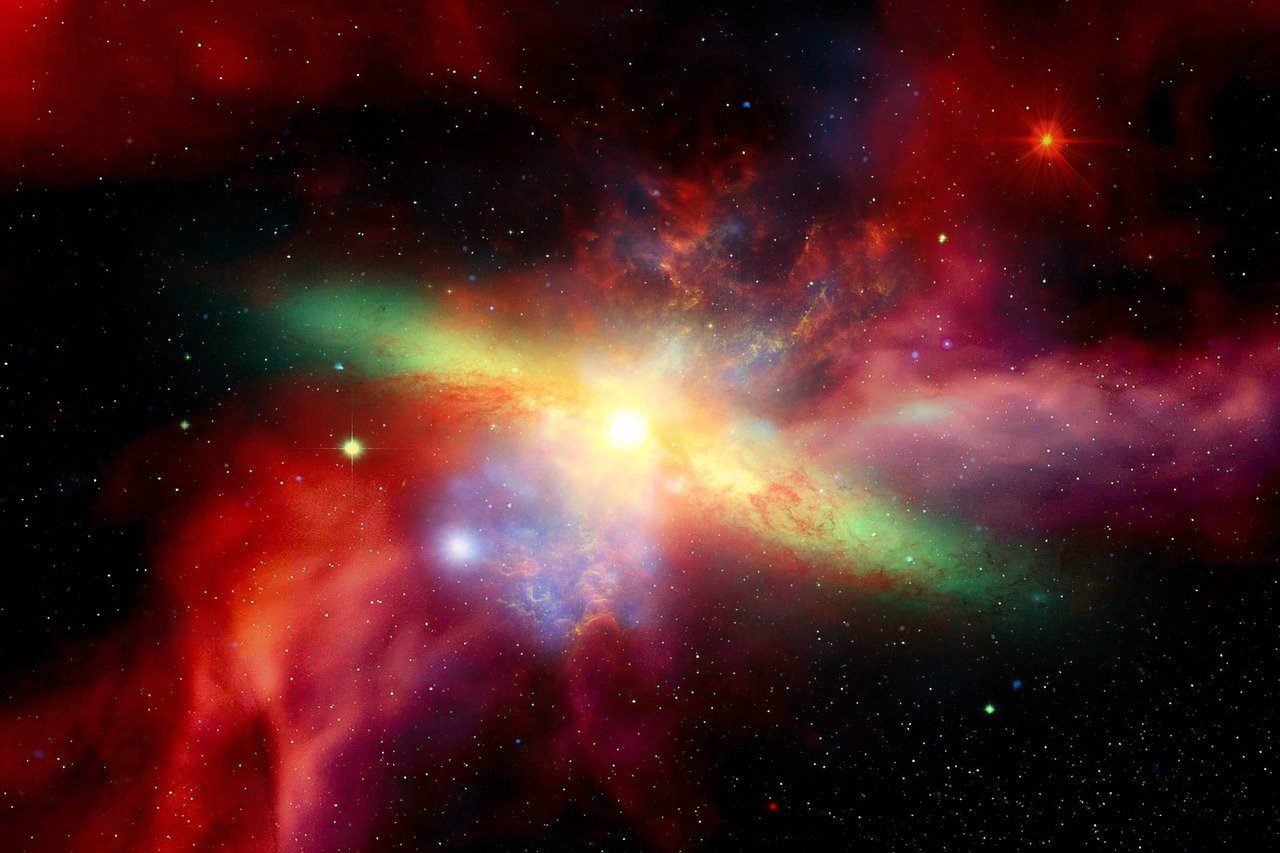
Advancements in Astrophysics
Astrophysics has been on a thrilling ride of discovery, and it’s not just the stars that are shining brighter; our understanding of the universe is expanding at an astonishing pace. Recent breakthroughs have reshaped our perspective on cosmic phenomena, leading us to question what we thought we knew about the cosmos. Imagine peering into a vast, dark ocean, where each wave reveals secrets of the universe—this is what modern astrophysics is doing for us!
One of the most significant advancements has been in the study of black holes. These enigmatic entities challenge our understanding of gravity and spacetime. For years, they were merely theoretical constructs, but now, thanks to groundbreaking research, we are starting to unravel their mysteries. The Event Horizon Telescope (EHT) has provided us with unprecedented images of black holes, which not only enhance our grasp of their properties but also confirm theoretical predictions made by Einstein's general relativity. This is akin to taking a photograph of a ghost that we only believed existed!
Black holes are not just cosmic vacuum cleaners; they are gateways to understanding the fundamental laws of the universe. When matter falls into a black hole, it reveals vital clues about the nature of singularities and the fabric of spacetime itself. The implications of such discoveries are profound, as they could lead to a new understanding of how the universe operates at its most fundamental level. As we continue to study these phenomena, we are not just observing; we are engaging in a dialogue with the universe.
The EHT, a network of radio telescopes around the globe, has allowed scientists to capture images of the event horizon—the boundary beyond which nothing can escape a black hole's gravitational pull. This achievement is like capturing the first-ever image of a distant planet; it opens a new chapter in our understanding of these cosmic giants. The images have confirmed that black holes are not just theoretical constructs, but real entities that play a crucial role in the evolution of galaxies. They are the engines that drive the cosmic machinery!
Another groundbreaking advancement in astrophysics is the detection of gravitational waves. These ripples in spacetime, predicted by Einstein over a century ago, were finally observed in 2015. The ability to detect gravitational waves has revolutionized our understanding of cosmic events such as colliding black holes and neutron stars. Imagine being able to hear the symphony of the universe—this is what gravitational wave astronomy offers us. It allows scientists to observe and analyze events that were previously invisible, reshaping our understanding of astrophysical phenomena.
With each new discovery, we are piecing together the cosmic puzzle, and the picture is more complex and beautiful than we ever imagined. The advancements in astrophysics are not just academic; they have real-world applications as well. Technologies developed for astrophysical research often find their way into everyday life, from improving communication systems to enhancing medical imaging techniques. The universe is not just a distant concept; it is intertwined with our very existence.
- What are black holes? Black holes are regions in space where the gravitational pull is so strong that nothing, not even light, can escape from them.
- How do we observe black holes? We observe black holes indirectly through their interactions with surrounding matter and through imaging techniques like those used by the Event Horizon Telescope.
- What are gravitational waves? Gravitational waves are ripples in spacetime caused by the acceleration of massive objects, such as merging black holes or neutron stars.
- Why are advancements in astrophysics important? They help us understand the fundamental laws of the universe, improve technology, and answer profound questions about our existence.
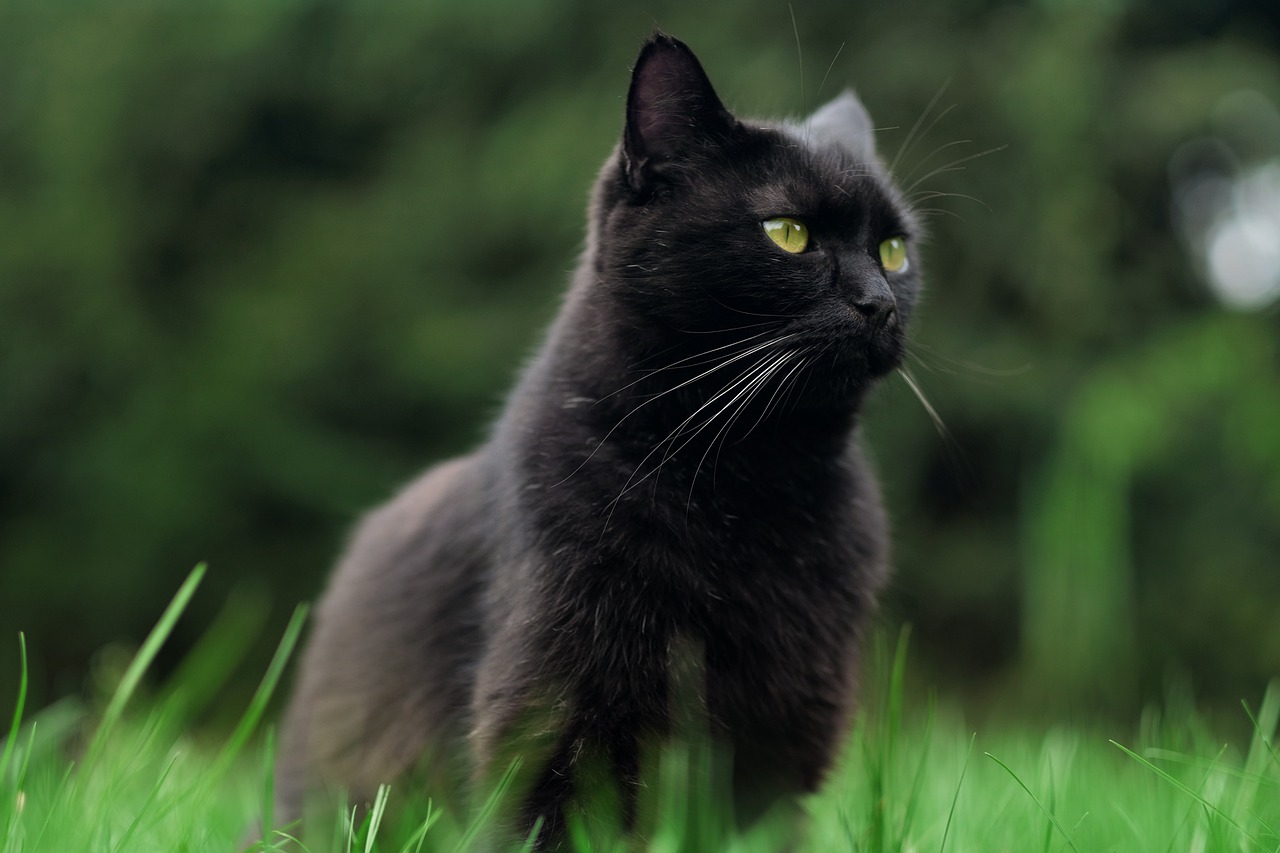
The Significance of Black Holes
Black holes are not just cosmic vacuum cleaners; they are the **gatekeepers of the universe's mysteries**. These enigmatic entities challenge our understanding of gravity and spacetime in ways that are both perplexing and fascinating. Imagine a region of space where the gravitational pull is so intense that nothing, not even light, can escape. This is the essence of a black hole, and it raises profound questions about the nature of reality itself.
At their core, black holes are formed when massive stars exhaust their nuclear fuel and collapse under their own gravity. This collapse leads to the formation of a singularity, a point where the laws of physics as we know them break down. The boundary surrounding this singularity is known as the event horizon. Crossing this threshold means that escape is impossible, making black holes a unique laboratory for testing the limits of our understanding of physics.
One of the most exciting aspects of black holes is their potential to unlock the secrets of the universe. Researchers are tirelessly working to answer questions such as:
- What happens to information that falls into a black hole?
- Can black holes provide insights into the fabric of spacetime?
- How do they influence the formation of galaxies?
These questions are not just academic; they touch on the very fabric of existence. The study of black holes could lead to groundbreaking discoveries that redefine our understanding of the universe. For instance, the **merging of black holes** has been detected through gravitational waves, providing a new way to observe cosmic events that were previously invisible. These observations have confirmed key aspects of Einstein's theory of general relativity, demonstrating that black holes are not just theoretical constructs but real, observable phenomena.
Moreover, black holes might hold the key to understanding **dark matter and dark energy**, two of the most significant components of our universe that remain largely mysterious. By studying the effects of black holes on surrounding matter and light, scientists hope to glean insights into these elusive forces that govern cosmic expansion and structure formation.
In summary, the significance of black holes extends far beyond their intimidating reputation. They are **pivotal players** in the cosmic drama, challenging our perceptions and pushing the boundaries of physics. As we continue to explore these fascinating objects, we may uncover truths that not only enhance our understanding of the universe but also illuminate the very nature of existence itself.
- What is a black hole? A black hole is a region in space where the gravitational pull is so strong that nothing, not even light, can escape from it.
- How are black holes formed? Black holes are formed when massive stars collapse under their own gravity after exhausting their nuclear fuel.
- Can we see black holes? While black holes themselves cannot be seen, their effects on nearby stars and gas can be observed, and recent advancements have allowed us to capture images of their event horizons.
- What are gravitational waves? Gravitational waves are ripples in spacetime caused by the acceleration of massive objects, such as colliding black holes, and were first detected in 2015.
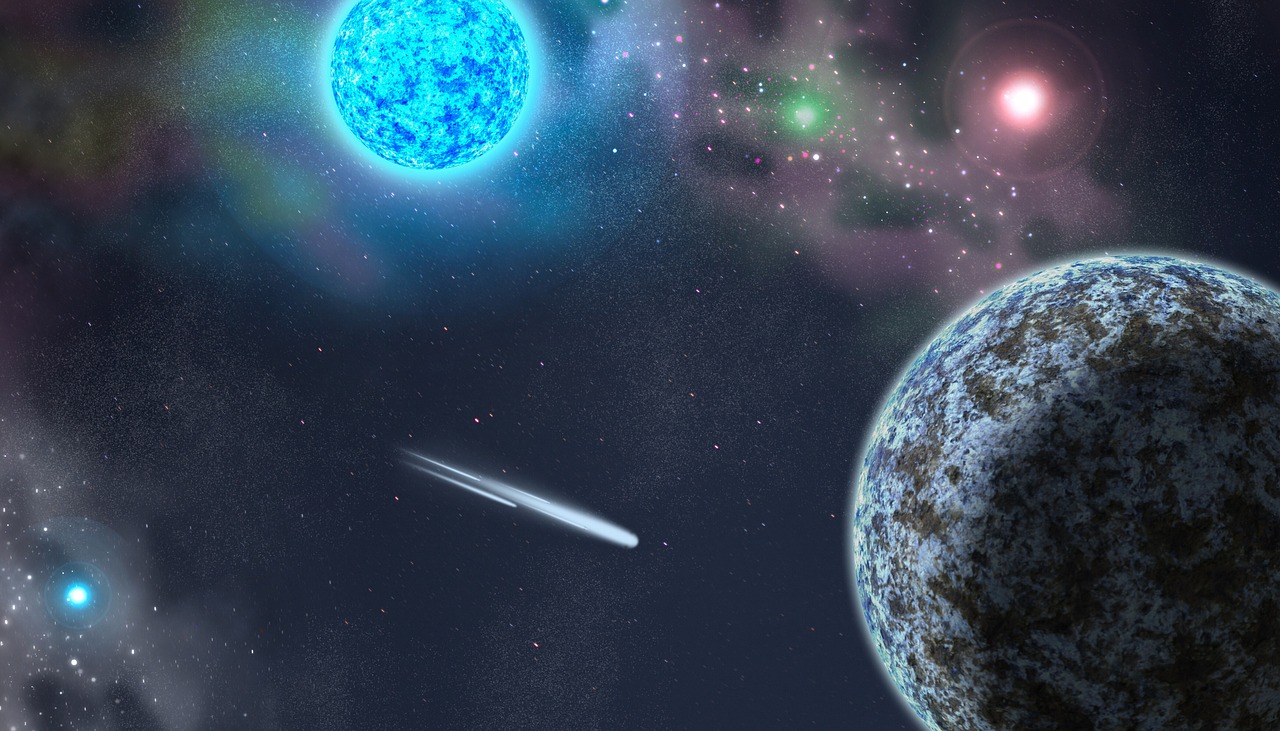
Event Horizon Telescope Discoveries
The Event Horizon Telescope (EHT) has been a game-changer in our quest to understand black holes, those enigmatic regions of spacetime where gravity is so intense that nothing, not even light, can escape. Launched in 2017, this global network of radio telescopes aimed to capture the first image of a black hole's event horizon, a feat that many considered impossible. However, in April 2019, the EHT team unveiled the stunning first image of the supermassive black hole at the center of the galaxy M87, which sent shockwaves through the scientific community and beyond.
This groundbreaking achievement not only provided visual evidence of black holes but also confirmed several predictions made by Albert Einstein's general relativity. The image revealed a bright ring of light surrounding a dark center, aptly named the "shadow" of the black hole. This shadow is a direct consequence of the black hole's gravitational pull bending light around it, showcasing the extreme warping of spacetime. The clarity and detail of the image were unprecedented, leading scientists to reevaluate existing theories and models of black hole behavior.
But the EHT's discoveries don't stop there. The collaboration has been instrumental in furthering our understanding of black hole properties, such as their mass and spin. For instance, the black hole in M87 was found to have a mass equivalent to about 6.5 billion suns. This finding is monumental, as it provides a benchmark for studying other black holes and their formation processes. Moreover, ongoing research using EHT data is delving into the dynamics of matter falling into black holes, known as accretion disks, and the jets of particles that are ejected from them at nearly the speed of light.
The EHT's work is not just about capturing images; it’s about unlocking the secrets of the universe. In 2022, the EHT collaboration released its first images of the black hole at the center of our own Milky Way galaxy, named Sagittarius A*. This discovery marked another significant milestone, providing a direct look at a black hole that is relatively close to us in cosmic terms. The implications of these findings are vast, as they help us understand how black holes grow and influence their surrounding environments. As researchers continue to analyze data from the EHT, we can expect even more revelations that could reshape our understanding of physics and the cosmos.
In summary, the Event Horizon Telescope has not only provided us with breathtaking images but has also confirmed long-held theories and opened new avenues of research. The discoveries made through this collaborative effort are paving the way for future explorations into the nature of black holes, their formation, and their role in the universe. As we stand on the brink of a new era in astrophysics, one can't help but wonder: what other secrets about our universe lie waiting to be uncovered?
- What is the Event Horizon Telescope?
The Event Horizon Telescope is a global network of radio telescopes that work together to observe black holes and capture images of their event horizons. - What did the EHT discover?
The EHT made history by capturing the first image of a black hole's event horizon, specifically in the galaxy M87, and later, the black hole at the center of the Milky Way. - Why are black holes important to study?
Studying black holes helps us understand fundamental aspects of physics, including gravity, spacetime, and the evolution of galaxies. - What are the implications of the EHT's discoveries?
The discoveries have confirmed predictions of general relativity and have opened new research avenues into the nature of black holes and their interactions with surrounding matter.
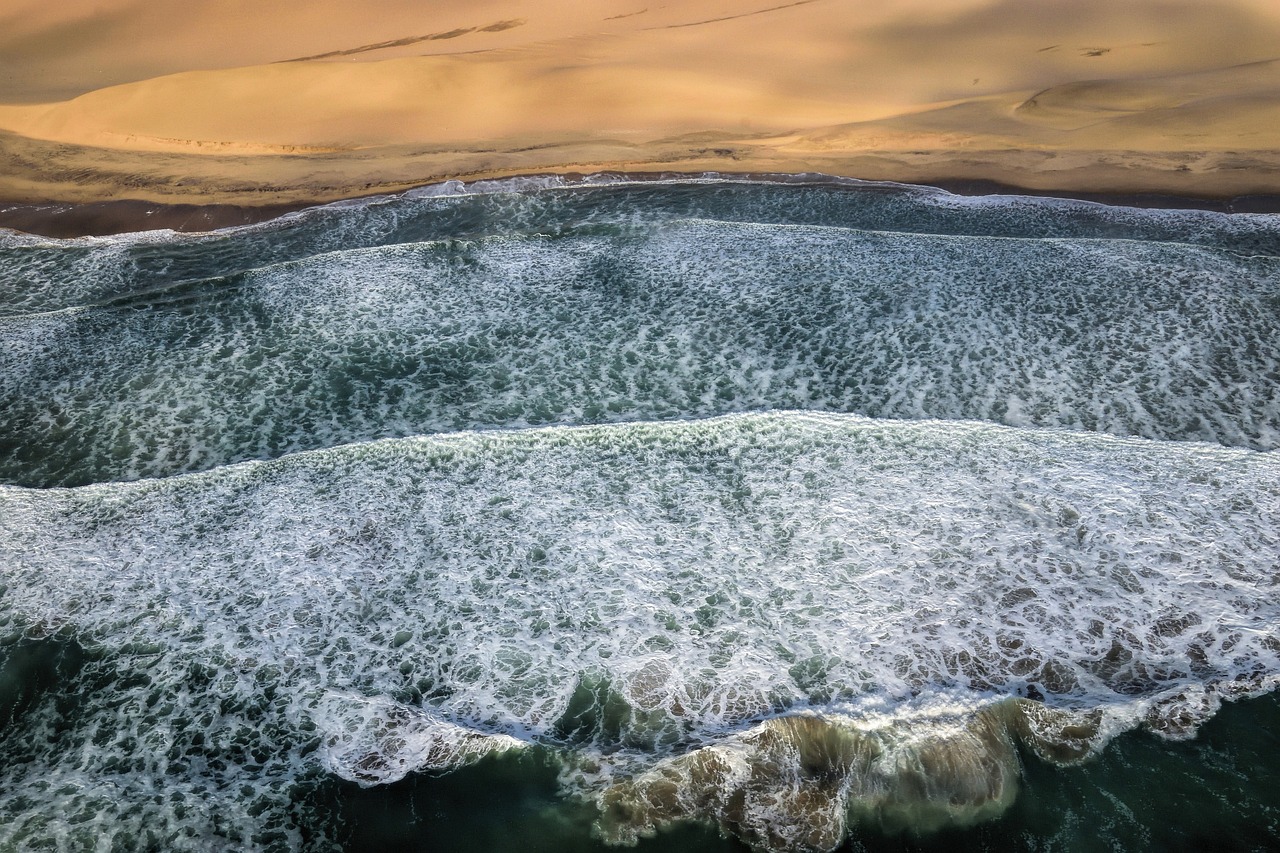
Gravitational Waves and Their Impact
Gravitational waves are ripples in spacetime caused by some of the most violent and energetic processes in the universe, such as the collision of black holes or neutron stars. When these massive celestial bodies merge, they create disturbances in the fabric of spacetime that travel across the universe at the speed of light. Imagine throwing a stone into a calm pond—the ripples that spread out are akin to gravitational waves, but instead of water, we’re dealing with the very fabric of reality itself. The detection of these waves has not only confirmed a key prediction of Einstein's general relativity but has also opened up a new avenue for astronomical observation.
Since the first detection by the LIGO (Laser Interferometer Gravitational-Wave Observatory) in 2015, the field has exploded with opportunities for researchers. Each detection provides a wealth of information about the sources of these waves and the fundamental physics behind them. For instance, scientists can now study the properties of black holes and neutron stars in ways that were previously unimaginable. This is akin to turning on a new sensory capability—suddenly, we can hear the universe in a way that was once thought to be impossible.
One of the most significant impacts of gravitational wave astronomy is its ability to complement traditional observations made through electromagnetic waves (like light). While telescopes allow us to observe the universe in various wavelengths, gravitational waves provide an entirely different perspective. They can reveal cosmic events that are invisible to optical telescopes, such as the merging of black holes. This multi-messenger astronomy enables scientists to piece together a more complete picture of cosmic events, leading to groundbreaking discoveries.
Furthermore, the implications of gravitational wave detection extend beyond mere observation. They challenge our understanding of fundamental physics, particularly in the realms of general relativity and quantum mechanics. As researchers delve deeper into the data, they may uncover new phenomena that could reshape our understanding of the universe. For example, the precise measurements of gravitational waves can test the limits of general relativity, potentially revealing discrepancies that could hint at new physics beyond our current models.
As we look to the future, the potential of gravitational wave astronomy is immense. Upcoming observatories, such as the space-based LISA (Laser Interferometer Space Antenna), are set to expand our capabilities further. By detecting lower-frequency gravitational waves, LISA will allow us to explore phenomena like supermassive black hole mergers and cosmic inflation, which occurred shortly after the Big Bang. The possibilities are tantalizing, and the discoveries yet to come could fundamentally alter our understanding of the cosmos.
In summary, gravitational waves are more than just a scientific curiosity; they are a powerful tool that is reshaping our understanding of the universe. By providing insights into the most extreme events in the cosmos, they not only confirm existing theories but also challenge us to rethink and expand our current understanding of physics.
- What are gravitational waves? Gravitational waves are ripples in spacetime caused by massive accelerating objects, such as colliding black holes or neutron stars.
- How are gravitational waves detected? They are detected using highly sensitive instruments like LIGO, which measures the minuscule changes in distance caused by passing gravitational waves.
- Why are gravitational waves important? They provide a new way to observe the universe, offering insights into cosmic events that are otherwise invisible to traditional telescopes.
- What future discoveries might gravitational waves lead to? Future observations could uncover new physics, enhance our understanding of black holes, and provide insights into the early universe.
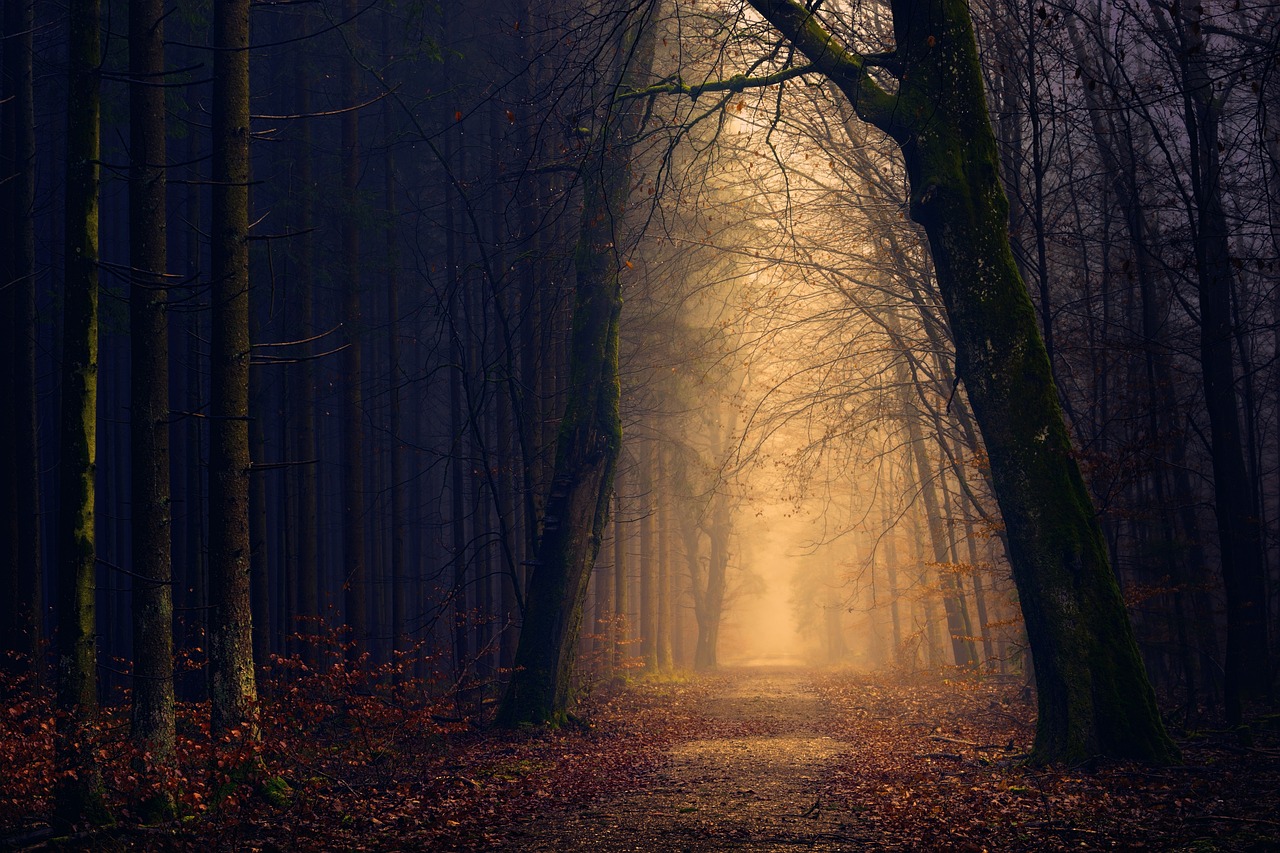
Dark Matter and Dark Energy
Imagine looking up at the night sky, filled with countless stars twinkling like diamonds against a vast, dark canvas. But what if I told you that most of what makes up our universe is invisible? This intriguing concept brings us to the realms of dark matter and dark energy, two of the most perplexing and significant components of our cosmic puzzle. Together, they account for about 95% of the total mass-energy content of the universe! Can you believe that? While we can observe ordinary matter—like stars, planets, and galaxies—dark matter and dark energy remain elusive, hidden from direct detection.
So, what exactly are these mysterious entities? Dark matter is thought to be a form of matter that does not emit, absorb, or reflect light, making it completely invisible and detectable only through its gravitational effects. It acts like a cosmic glue, holding galaxies together and influencing their rotation. Without dark matter, galaxies would fly apart, unable to maintain their structure. Scientists estimate that dark matter constitutes about 27% of the universe's total mass-energy content.
On the other hand, we have dark energy, which is an even more enigmatic force. It is believed to be responsible for the accelerated expansion of the universe. While dark matter pulls things together, dark energy pushes them apart. This counterintuitive behavior raises fascinating questions about the fate of the universe. Will it continue to expand forever? Or will it eventually collapse? Currently, dark energy is thought to make up about 68% of the universe's total mass-energy content.
To better understand these concepts, let’s take a look at the following table summarizing dark matter and dark energy:
Component | Percentage of Universe | Characteristics |
---|---|---|
Dark Matter | 27% | Invisible, interacts through gravity, holds galaxies together |
Dark Energy | 68% | Invisible, causes accelerated expansion of the universe |
The quest to unravel the mysteries of dark matter and dark energy is ongoing. Scientists are employing various methods, from advanced telescopes to particle accelerators, to detect and study these phenomena. For instance, experiments like the Large Hadron Collider (LHC) aim to create conditions that might reveal dark matter particles. Meanwhile, observatories are mapping the universe's expansion to gain insights into dark energy's properties.
In summary, dark matter and dark energy are not just scientific curiosities; they are crucial to our understanding of the universe's structure and evolution. As researchers continue to delve deeper into these mysteries, we may find answers that not only change our perception of the cosmos but also redefine our place within it. Isn't it thrilling to think about what lies beyond our current understanding? The universe is full of surprises, and as we push the boundaries of knowledge, who knows what revelations await us?
- What is dark matter? Dark matter is a type of matter that does not emit light or energy, making it invisible. It is detected through its gravitational effects on visible matter.
- How does dark energy affect the universe? Dark energy is believed to be responsible for the accelerated expansion of the universe, counteracting the force of gravity.
- Why is dark matter important? Dark matter plays a crucial role in the formation and structure of galaxies, influencing how they hold together and evolve over time.
- Can dark matter and dark energy be observed directly? Currently, dark matter and dark energy cannot be observed directly; scientists study their effects on visible matter and the universe's expansion.
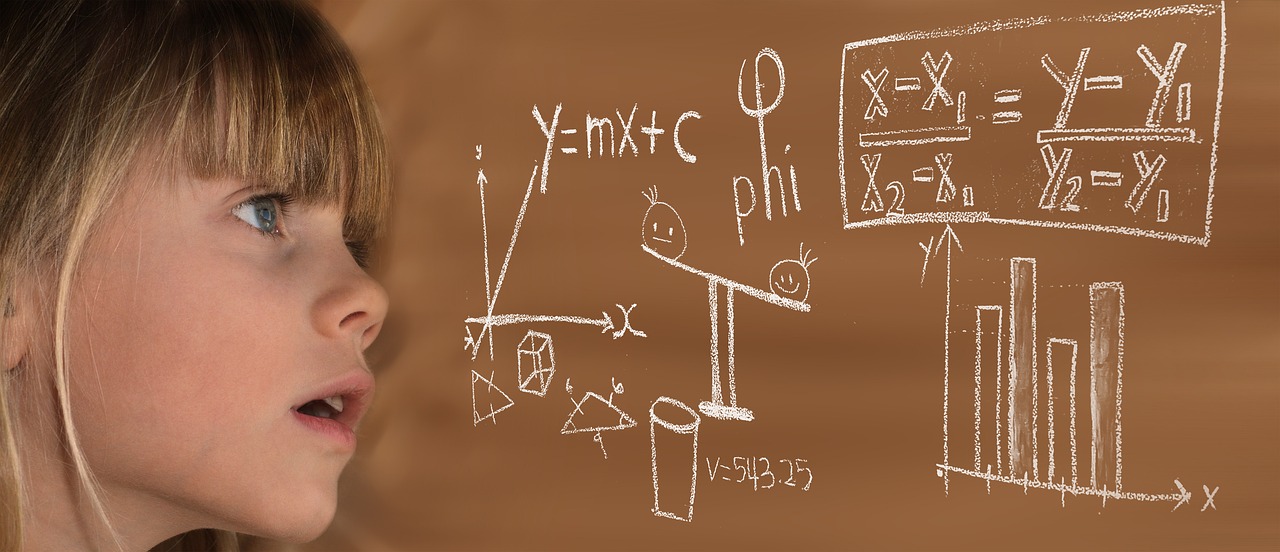
The Standard Model of Particle Physics
The Standard Model of Particle Physics is often regarded as the cornerstone of modern physics. It describes the fundamental particles that make up our universe and the forces that govern their interactions. Think of it as a comprehensive recipe book that details the ingredients (particles) and the methods (forces) used to create the universe as we know it. However, just like any good recipe, new discoveries can lead to modifications and improvements, and that's precisely what we're witnessing in the field of particle physics today.
At its core, the Standard Model categorizes particles into two main groups: fermions and bosons. Fermions are the building blocks of matter, including quarks and leptons, while bosons are responsible for mediating forces between fermions. The most famous boson, the Higgs boson, was discovered in 2012 and confirmed the existence of the Higgs field, which gives mass to particles. This discovery was monumental, akin to finding the missing piece of a jigsaw puzzle; it completed our understanding of how particles acquire mass and solidified the Standard Model's validity.
Despite its success, the Standard Model is not without its limitations. For instance, it does not account for gravity, nor does it explain the mysterious components of the universe such as dark matter and dark energy. These gaps have led physicists to ponder the possibility of theories that extend beyond the Standard Model. This pursuit for a more comprehensive understanding can be likened to explorers venturing into uncharted territories, seeking new lands that may hold the key to the universe's most profound mysteries.
To illustrate the components of the Standard Model, we can break it down into a simple table:
Particle Type | Examples | Role |
---|---|---|
Fermions | Quarks, Leptons | Building blocks of matter |
Bosons | Photon, W and Z bosons, Gluon, Higgs boson | Mediators of forces |
As we delve deeper into the fabric of the universe, physicists are exploring theories that could potentially unify the forces described in the Standard Model. Concepts like supersymmetry and string theory are at the forefront of this research, aiming to answer questions that remain unanswered. These theories propose that there may be additional particles and dimensions that we have yet to discover, which could fundamentally alter our understanding of the universe.
In conclusion, while the Standard Model of Particle Physics has provided us with invaluable insights into the workings of the universe, it is merely a stepping stone towards a more profound understanding. The quest for knowledge continues, driven by curiosity and the desire to uncover the secrets that lie beyond our current grasp. As we venture into this new frontier, who knows what astonishing discoveries await us?
- What is the Standard Model of Particle Physics? - It is a theory that describes the fundamental particles and forces that make up the universe.
- Why is the Higgs boson important? - The Higgs boson confirms the mechanism that gives mass to particles, which is crucial for understanding the universe's structure.
- What are dark matter and dark energy? - They are mysterious components of the universe that the Standard Model does not explain, prompting further research.
- What theories go beyond the Standard Model? - Theories like supersymmetry and string theory aim to address the gaps in the Standard Model and unify the forces of nature.
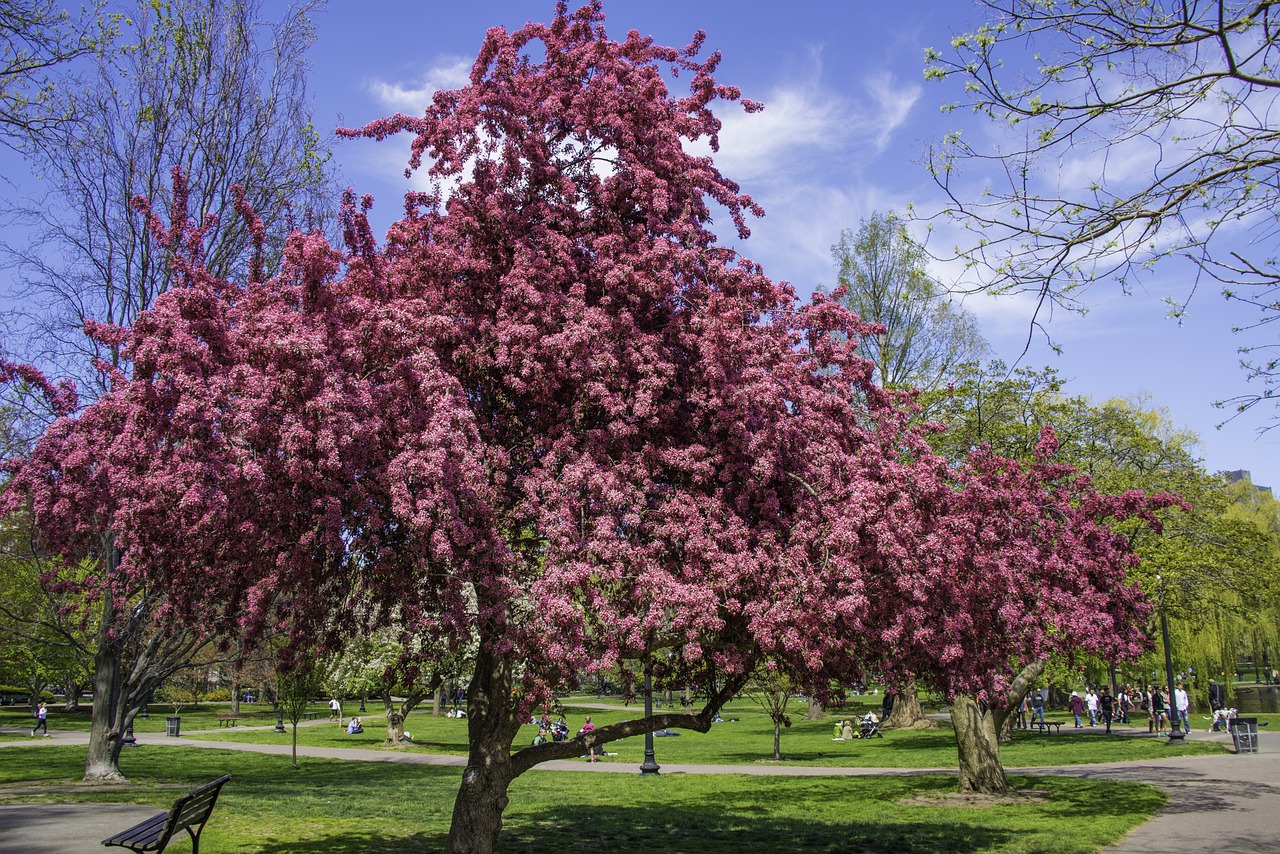
Higgs Boson Discovery
The discovery of the Higgs boson in 2012 at CERN's Large Hadron Collider was nothing short of a scientific revolution. This elusive particle, often referred to as the "God particle," plays a crucial role in our understanding of the universe. It is the key to the Higgs field, a fundamental field that permeates all of space and gives mass to elementary particles. Imagine the Higgs field as a thick syrup spread throughout the cosmos; particles that interact with this field acquire mass, while those that do not remain massless, much like a person trying to run through molasses versus one gliding effortlessly on ice.
Before its discovery, the existence of the Higgs boson was merely a theoretical prediction, integral to the Standard Model of particle physics. This model describes the fundamental particles and their interactions, but it was incomplete without the Higgs boson. The particle's discovery confirmed the last missing piece of the puzzle, solidifying our understanding of how particles obtain mass. The implications of this discovery extend far beyond particle physics; they touch on the very fabric of the universe itself.
To grasp the significance of the Higgs boson, one must understand its implications on the fundamental forces of nature. The Higgs mechanism explains why some particles have mass while others, like photons, do not. This distinction is vital because mass influences how particles interact with one another and how they form the matter that makes up stars, planets, and ultimately, us. Without the Higgs boson, the universe would be a vastly different place—one where atoms could not form, and life as we know it would be impossible.
Since the Higgs boson's discovery, scientists have been tirelessly working to study its properties. They aim to answer critical questions such as:
- What is the exact mass of the Higgs boson?
- How does it interact with other particles?
- Are there multiple types of Higgs bosons?
These questions are not just academic; they could lead to breakthroughs in our understanding of the universe's fundamental laws. Researchers are also exploring the possibility of new physics beyond the Standard Model, which could reveal even more about the nature of reality. For instance, if the Higgs boson has additional properties or if there are other, yet-to-be-discovered particles, it could reshape our understanding of the universe.
In summary, the discovery of the Higgs boson is a monumental achievement in physics, validating decades of theoretical work and opening new avenues for exploration. As we delve deeper into the mysteries of this particle, we inch closer to answering some of the most profound questions about the universe and our place within it.
- What is the Higgs boson? The Higgs boson is a fundamental particle associated with the Higgs field, responsible for giving mass to other elementary particles.
- Why is the Higgs boson called the "God particle"? The nickname "God particle" reflects the particle's essential role in the universe, though many scientists prefer to avoid this term due to its sensational connotations.
- How was the Higgs boson discovered? The Higgs boson was discovered through experiments at the Large Hadron Collider, where scientists collided protons at high energies and analyzed the resulting particle interactions.
- What are the implications of the Higgs boson discovery? The discovery confirmed the Standard Model of particle physics and has significant implications for our understanding of mass, fundamental forces, and the universe's structure.
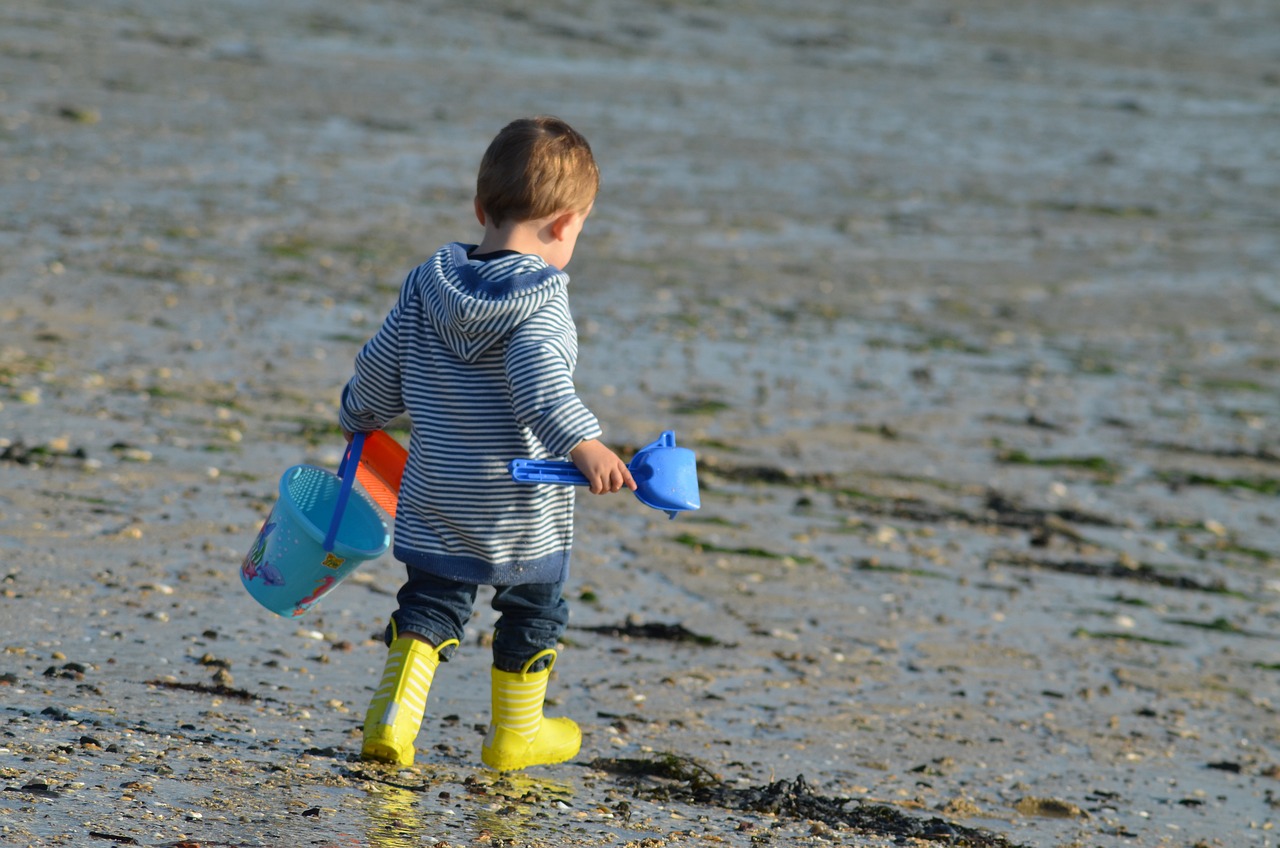
Beyond the Standard Model
The quest to understand the universe has always pushed scientists to explore uncharted territories, and one of the most intriguing areas of research today is what lies of particle physics. The Standard Model has been a cornerstone of modern physics, successfully describing the fundamental particles and forces that govern the universe. However, it has its limitations and unanswered questions, which have led physicists to seek new theories that could provide a more comprehensive understanding of the cosmos.
One of the main motivations for looking beyond the Standard Model is the existence of phenomena that it cannot adequately explain. For instance, the mystery of dark matter and dark energy poses significant challenges. These components make up about 95% of the universe, yet they remain elusive and unaccounted for in the Standard Model. This has led to the exploration of various theories, including supersymmetry, which suggests that every particle has a superpartner, potentially providing a solution to the dark matter conundrum.
Another promising avenue of research is string theory, which posits that the fundamental building blocks of the universe are not point-like particles but rather tiny, vibrating strings. This theory aims to unify all fundamental forces, including gravity, into a single framework. While string theory is still in its infancy and remains largely theoretical, it has the potential to bridge gaps in our understanding, offering insights into the fabric of space-time itself.
Moreover, the exploration of quantum gravity seeks to reconcile the principles of quantum mechanics with general relativity, which governs the behavior of large-scale structures in the universe. This endeavor is crucial for understanding phenomena such as black holes and the Big Bang, where both quantum effects and gravitational forces are at play. Theoretical physicists are actively working on models that could provide a coherent description of gravity at quantum scales, potentially leading to groundbreaking discoveries.
In summary, the pursuit of knowledge beyond the Standard Model is not just an academic exercise; it is a vital part of our quest to understand the universe. As we delve deeper into these theories, we may uncover new particles, forces, and even dimensions that challenge our current understanding and expand the horizons of physics. The journey is fraught with challenges, but the potential rewards are immense, promising to reshape our view of reality itself.
- What is the Standard Model of particle physics?
The Standard Model is a theoretical framework that describes the fundamental particles and forces in the universe, including quarks, leptons, and the forces of electromagnetism, weak nuclear force, and strong nuclear force. - Why do scientists look beyond the Standard Model?
Scientists look beyond the Standard Model to address unanswered questions, such as the nature of dark matter and dark energy, and to explore new theories that could unify all fundamental forces. - What is supersymmetry?
Supersymmetry is a proposed extension of the Standard Model that suggests every particle has a superpartner, potentially providing insights into dark matter and other phenomena. - What is string theory?
String theory is a theoretical framework that posits that the fundamental building blocks of the universe are tiny, vibrating strings, aiming to unify all fundamental forces, including gravity. - What is quantum gravity?
Quantum gravity is an area of research that seeks to reconcile the principles of quantum mechanics with general relativity, particularly in extreme conditions like black holes and the early universe.
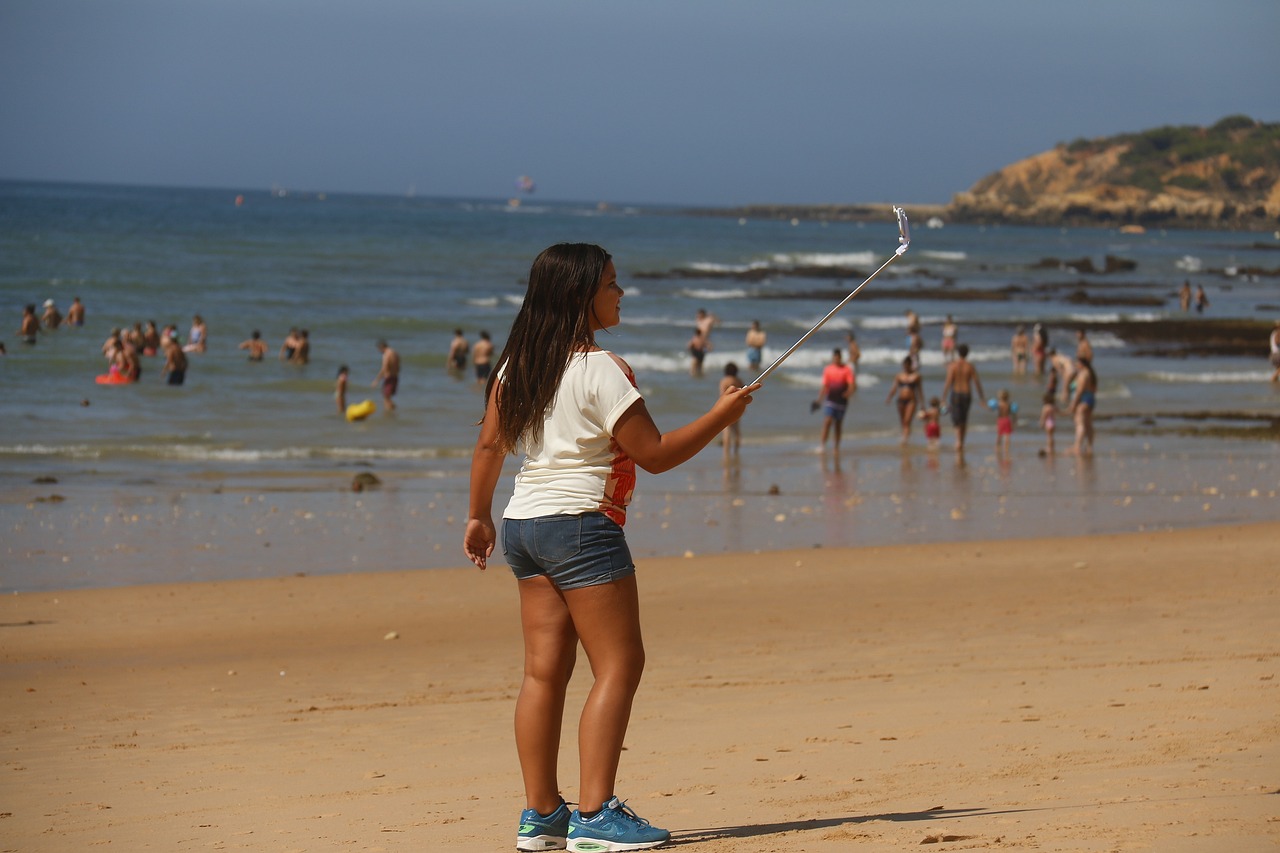
Future of Theoretical Physics
The future of theoretical physics is a vast and exciting frontier, filled with possibilities that could forever alter our understanding of the universe. As we stand on the cusp of new discoveries, the questions we ask today will shape the research of tomorrow. Imagine a world where the mysteries of quantum gravity are unraveled, where we can finally understand how the forces of gravity interact with the quantum realm. This is not just a dream; it's a tantalizing possibility that researchers are actively pursuing.
One of the most intriguing areas of exploration is the concept of the multiverse. What if our universe is just one of many? The idea that there could be countless universes, each with its own set of physical laws, challenges our fundamental grasp of reality. This notion isn't just science fiction; it's a serious hypothesis that physicists are beginning to explore. The implications of such a discovery would be monumental. It could redefine our understanding of existence itself, leading to profound philosophical questions: What does it mean to be 'alive' in a multiverse?
Another exciting avenue is the pursuit of a unified theory that combines all fundamental forces of nature. Currently, we have separate theories for gravity, electromagnetism, and nuclear forces, but a theory of everything could bridge these gaps. This quest could lead to revolutionary insights into the fabric of reality, potentially revealing new particles or forces yet to be discovered. For instance, theories like string theory and loop quantum gravity are at the forefront of this research, offering different perspectives on how to unify these forces.
As we delve deeper into these theories, the role of advanced technology cannot be overstated. The development of more sophisticated particle accelerators and telescopes will enhance our ability to test these theories. For example, the next generation of particle colliders could provide evidence for supersymmetry or other new physics beyond the Standard Model. With each experiment, we inch closer to answering the big questions that have puzzled humanity for centuries.
Moreover, the intersection of artificial intelligence and physics is another area ripe for exploration. AI has the potential to analyze vast quantities of data far beyond human capability, identifying patterns and anomalies that could lead to groundbreaking discoveries. Imagine a future where AI collaborates with physicists to uncover the secrets of the universe, accelerating the pace of research exponentially.
In conclusion, the future of theoretical physics is not just about answering existing questions; it's about asking new ones. As we push the boundaries of our understanding, we may find that the universe is far more complex and beautiful than we ever imagined. With each new discovery, we take a step closer to understanding our place in this vast cosmos. The journey is just beginning, and the possibilities are as limitless as the universe itself.
- What is theoretical physics?
Theoretical physics is a branch of physics that uses mathematical models and abstractions to explain and predict physical phenomena. - Why is the study of quantum gravity important?
Quantum gravity aims to reconcile general relativity and quantum mechanics, potentially leading to a more comprehensive understanding of the universe. - What is the multiverse theory?
The multiverse theory suggests that there could be multiple, perhaps infinite, universes that exist alongside our own, each with different physical laws. - How does AI contribute to physics research?
AI can process and analyze large datasets, helping physicists identify trends and make predictions that would be difficult to achieve manually.
Frequently Asked Questions
- What is quantum mechanics and why is it important?
Quantum mechanics is a fundamental theory in physics that describes the behavior of matter and energy at the smallest scales, such as atoms and subatomic particles. Its importance lies in its ability to explain phenomena that classical physics cannot, leading to revolutionary technologies like quantum computing and cryptography.
- How have black holes changed our understanding of the universe?
Black holes challenge traditional notions of gravity and spacetime, revealing the extreme conditions that exist in the universe. They have become a focal point for research, helping scientists explore the laws governing the cosmos and the nature of singularities.
- What are gravitational waves and why are they significant?
Gravitational waves are ripples in spacetime caused by massive cosmic events, such as colliding black holes or neutron stars. Their detection has transformed astrophysics, allowing scientists to observe and understand these phenomena in ways that were previously impossible.
- What is dark matter and dark energy?
Dark matter and dark energy are mysterious components of the universe that make up about 95% of its total mass-energy content. While dark matter helps explain the gravitational effects observed in galaxies, dark energy is thought to drive the accelerated expansion of the universe.
- What is the Standard Model of particle physics?
The Standard Model is a well-established theory that describes the fundamental particles and forces that make up the universe. It has been successful in explaining a wide range of phenomena, but recent discoveries have raised questions about its completeness, leading physicists to explore theories beyond it.
- What was the significance of the Higgs boson discovery?
The discovery of the Higgs boson was a groundbreaking achievement in physics, confirming the mechanism that gives mass to elementary particles. This discovery not only validated the Standard Model but also opened new avenues for research into the fundamental structure of the universe.
- What are some theories beyond the Standard Model?
Theories beyond the Standard Model include supersymmetry and string theory, which aim to address unresolved questions in physics. These theories seek to provide a more unified understanding of the universe by proposing new particles and dimensions beyond our current knowledge.
- What does the future hold for theoretical physics?
The future of theoretical physics is promising, with ongoing research into topics like quantum gravity, multiverse theories, and the nature of time itself. These explorations could redefine our understanding of the universe and lead to groundbreaking discoveries.